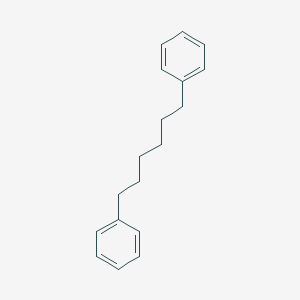
1,6-Diphenylhexane
Overview
Description
1,6-Diphenylhexane is an organic compound with the molecular formula C₁₈H₂₂. It consists of a hexane chain with phenyl groups attached to the first and sixth carbon atoms. This compound is known for its structural simplicity and stability, making it a subject of interest in various chemical studies .
Mechanism of Action
Target of Action
1,6-Diphenylhexane is a complex organic compound with the molecular formula C18H22 One study suggests that it may interact with a protein called snt2 .
Mode of Action
It has been suggested that this compound-1,3,4,6-tetrone shows a high docking energy against the target protein snt2, which may result in the downregulation of the protein’s expression .
Pharmacokinetics
Its molecular weight is 23837 , which may influence its absorption and distribution in the body
Result of Action
It has been suggested that it may downregulate the expression of the protein snt2 .
Action Environment
Factors such as temperature and ph often play a role in the action of organic compounds .
Preparation Methods
Synthetic Routes and Reaction Conditions
1,6-Diphenylhexane can be synthesized through several methods. One common approach involves the Friedel-Crafts alkylation reaction, where benzene reacts with 1,6-dibromohexane in the presence of a Lewis acid catalyst such as aluminum chloride (AlCl₃). The reaction proceeds under anhydrous conditions and typically requires refluxing the mixture for several hours .
Industrial Production Methods
Industrial production of this compound often employs similar Friedel-Crafts alkylation techniques but on a larger scale. The process involves rigorous control of reaction conditions, including temperature, pressure, and catalyst concentration, to ensure high yield and purity of the final product .
Chemical Reactions Analysis
Types of Reactions
1,6-Diphenylhexane undergoes various chemical reactions, including:
Oxidation: This compound can be oxidized to form this compound-1,6-dione using strong oxidizing agents such as potassium permanganate (KMnO₄) or chromium trioxide (CrO₃).
Reduction: Reduction of this compound-1,6-dione can yield this compound-1,6-diol using reducing agents like sodium borohydride (NaBH₄).
Common Reagents and Conditions
Oxidation: Potassium permanganate (KMnO₄) in acidic or neutral medium.
Reduction: Sodium borohydride (NaBH₄) in methanol or ethanol.
Substitution: Bromine (Br₂) or chlorine (Cl₂) in the presence of UV light or a catalyst.
Major Products Formed
Oxidation: this compound-1,6-dione.
Reduction: this compound-1,6-diol.
Substitution: Halogenated derivatives of this compound.
Scientific Research Applications
1,6-Diphenylhexane has diverse applications in scientific research:
Chemistry: It is used as a model compound in studies of hydrocarbon behavior and reaction mechanisms.
Biology: Research into its interactions with biological membranes and its potential as a hydrophobic probe.
Medicine: Investigated for its potential use in drug delivery systems due to its hydrophobic nature.
Comparison with Similar Compounds
Similar Compounds
1,6-Diphenylhexane-1,6-dione: An oxidized form of this compound.
This compound-1,6-diol: A reduced form of this compound.
1,6-Dibromohexane: A precursor used in the synthesis of this compound
Uniqueness
This compound is unique due to its structural simplicity and stability, which make it an ideal model compound for studying hydrocarbon behavior and reaction mechanisms. Its ability to undergo various chemical reactions, including oxidation, reduction, and substitution, further enhances its versatility in scientific research .
Properties
IUPAC Name |
6-phenylhexylbenzene | |
---|---|---|
Source | PubChem | |
URL | https://pubchem.ncbi.nlm.nih.gov | |
Description | Data deposited in or computed by PubChem | |
InChI |
InChI=1S/C18H22/c1(5-11-17-13-7-3-8-14-17)2-6-12-18-15-9-4-10-16-18/h3-4,7-10,13-16H,1-2,5-6,11-12H2 | |
Source | PubChem | |
URL | https://pubchem.ncbi.nlm.nih.gov | |
Description | Data deposited in or computed by PubChem | |
InChI Key |
DVGPMIRVCZNXMD-UHFFFAOYSA-N | |
Source | PubChem | |
URL | https://pubchem.ncbi.nlm.nih.gov | |
Description | Data deposited in or computed by PubChem | |
Canonical SMILES |
C1=CC=C(C=C1)CCCCCCC2=CC=CC=C2 | |
Source | PubChem | |
URL | https://pubchem.ncbi.nlm.nih.gov | |
Description | Data deposited in or computed by PubChem | |
Molecular Formula |
C18H22 | |
Source | PubChem | |
URL | https://pubchem.ncbi.nlm.nih.gov | |
Description | Data deposited in or computed by PubChem | |
DSSTOX Substance ID |
DTXSID80148717 | |
Record name | 1,6-Diphenylhexane | |
Source | EPA DSSTox | |
URL | https://comptox.epa.gov/dashboard/DTXSID80148717 | |
Description | DSSTox provides a high quality public chemistry resource for supporting improved predictive toxicology. | |
Molecular Weight |
238.4 g/mol | |
Source | PubChem | |
URL | https://pubchem.ncbi.nlm.nih.gov | |
Description | Data deposited in or computed by PubChem | |
CAS No. |
1087-49-6 | |
Record name | 1,6-Diphenylhexane | |
Source | ChemIDplus | |
URL | https://pubchem.ncbi.nlm.nih.gov/substance/?source=chemidplus&sourceid=0001087496 | |
Description | ChemIDplus is a free, web search system that provides access to the structure and nomenclature authority files used for the identification of chemical substances cited in National Library of Medicine (NLM) databases, including the TOXNET system. | |
Record name | 1,6-Diphenylhexane | |
Source | DTP/NCI | |
URL | https://dtp.cancer.gov/dtpstandard/servlet/dwindex?searchtype=NSC&outputformat=html&searchlist=44143 | |
Description | The NCI Development Therapeutics Program (DTP) provides services and resources to the academic and private-sector research communities worldwide to facilitate the discovery and development of new cancer therapeutic agents. | |
Explanation | Unless otherwise indicated, all text within NCI products is free of copyright and may be reused without our permission. Credit the National Cancer Institute as the source. | |
Record name | 1,6-Diphenylhexane | |
Source | EPA DSSTox | |
URL | https://comptox.epa.gov/dashboard/DTXSID80148717 | |
Description | DSSTox provides a high quality public chemistry resource for supporting improved predictive toxicology. | |
Synthesis routes and methods
Procedure details
Retrosynthesis Analysis
AI-Powered Synthesis Planning: Our tool employs the Template_relevance Pistachio, Template_relevance Bkms_metabolic, Template_relevance Pistachio_ringbreaker, Template_relevance Reaxys, Template_relevance Reaxys_biocatalysis model, leveraging a vast database of chemical reactions to predict feasible synthetic routes.
One-Step Synthesis Focus: Specifically designed for one-step synthesis, it provides concise and direct routes for your target compounds, streamlining the synthesis process.
Accurate Predictions: Utilizing the extensive PISTACHIO, BKMS_METABOLIC, PISTACHIO_RINGBREAKER, REAXYS, REAXYS_BIOCATALYSIS database, our tool offers high-accuracy predictions, reflecting the latest in chemical research and data.
Strategy Settings
Precursor scoring | Relevance Heuristic |
---|---|
Min. plausibility | 0.01 |
Model | Template_relevance |
Template Set | Pistachio/Bkms_metabolic/Pistachio_ringbreaker/Reaxys/Reaxys_biocatalysis |
Top-N result to add to graph | 6 |
Feasible Synthetic Routes
Q1: What is the structural characterization of 1,6-diphenylhexane?
A1: this compound is an alkane with two phenyl groups attached to the terminal carbons of a six-carbon chain.
- Spectroscopic Data: While specific data depends on the isomer and experimental conditions, studies have investigated the gas-phase protonation of this compound using FT-ICR mass spectrometry. []
Q2: How does the structure of this compound relate to its gas-phase basicity?
A2: Research shows that the gas-phase basicity (GB) of this compound is higher than toluene due to increased polarizability. [] Interestingly, the GB of α,ω-diphenylalkanes, including this compound, varies with the length of the methylene chain connecting the phenyl rings. This difference is attributed to intramolecular solvation of the protonated phenyl ring by the neutral one, enabled by specific conformations of the linking chain. []
Q3: Has this compound been used in the synthesis of any significant pharmaceutical compounds?
A3: Yes, derivatives of this compound are crucial building blocks for synthesizing HIV protease inhibitors. For instance, (2S,3S,5S)-2,5-diamino-3-hydroxy-1,6-diphenylhexane serves as a key intermediate in the synthesis of lopinavir, a medication used to treat HIV. [, ]
Q4: Can you elaborate on the synthesis of (2S,3S,5S)-2,5-diamino-3-hydroxy-1,6-diphenylhexane?
A4: Several methods have been explored for the synthesis of this important intermediate. One approach utilizes L-phenylalanine as the starting material, subjecting it to N,O-benzylation, cyanidation, Grignard reaction, and reduction to yield the desired compound. [] Another method involves a multi-step process starting with the protection of L-phenylalanine with benzyl chloride, followed by reactions with acetonitrile and benzylmagnesium chloride, and finally, reduction with sodium borohydride. []
Q5: What is the significance of the (2S,3S,5S)-stereochemistry in these pharmaceutical applications?
A5: The (2S,3S,5S)-stereoisomer of the diamino alcohol is crucial for the biological activity of HIV protease inhibitors like lopinavir. This specific stereoisomer exhibits optimal binding affinity to the active site of the HIV protease enzyme, allowing it to effectively inhibit viral replication. [, ]
Q6: Have there been any studies exploring modifications to the this compound core for improved drug efficacy?
A6: Yes, researchers have explored incorporating carbazole into the core structure of ritonavir, another HIV protease inhibitor, by modifying the (2S, 3S, 5S)-5-(tert-butyloxycarbonyl)amino-2-amino-3-hydroxy-1,6-diphenylhexane moiety. This modification aimed to improve the drug's solubility and potentially enhance its pharmacokinetic properties. []
Q7: Are there any studies focusing on the environmental impact of this compound and its derivatives?
A7: While the provided research focuses on the synthesis and applications of this compound and its derivatives, information regarding their environmental impact or degradation pathways is limited. Further research is needed to assess their potential ecotoxicological effects and develop strategies for responsible waste management and mitigation of any negative impacts.
Q8: How do ordered media like liquid crystals influence the photochemistry of diketone derivatives of this compound?
A8: Studies utilizing liquid crystals as solvents have investigated the Norrish Type II photoreactions of this compound-3,4-dione. [] Results indicate that the quantum yield for the disappearance of this diketone is influenced by both temperature and the order of the surrounding media. Interestingly, the quantum yield ratio for its disappearance in cholesteric mesophases and hexane is less than one, suggesting an impact of solvent order on the solute's conformational flexibility. []
Q9: Have there been investigations into the crystal structure of this compound derivatives?
A9: Yes, the crystal structure of this compound-1,3,4,6-tetrone has been determined. [] This research builds upon previous studies investigating the crystal structure of 1,3,5-triketone derivatives and employs techniques like NMR, IR, and mass spectrometry to explore the equilibrium between their keto and enol forms. []
Disclaimer and Information on In-Vitro Research Products
Please be aware that all articles and product information presented on BenchChem are intended solely for informational purposes. The products available for purchase on BenchChem are specifically designed for in-vitro studies, which are conducted outside of living organisms. In-vitro studies, derived from the Latin term "in glass," involve experiments performed in controlled laboratory settings using cells or tissues. It is important to note that these products are not categorized as medicines or drugs, and they have not received approval from the FDA for the prevention, treatment, or cure of any medical condition, ailment, or disease. We must emphasize that any form of bodily introduction of these products into humans or animals is strictly prohibited by law. It is essential to adhere to these guidelines to ensure compliance with legal and ethical standards in research and experimentation.