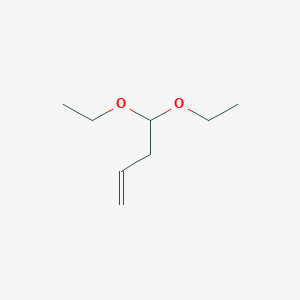
4,4-Diethoxybut-1-ene
Overview
Description
4,4-Diethoxybut-1-ene is an organic compound with the molecular formula C8H16O2 It is a derivative of butene, where two ethoxy groups are attached to the fourth carbon atom
Preparation Methods
Synthetic Routes and Reaction Conditions
4,4-Diethoxybut-1-ene can be synthesized through the reaction of but-1-ene with diethyl ether in the presence of a strong acid catalyst. The reaction typically involves the following steps:
Formation of the carbocation: The but-1-ene undergoes protonation by the acid catalyst, forming a carbocation intermediate.
Nucleophilic attack: The carbocation is then attacked by diethyl ether, leading to the formation of this compound.
The reaction conditions generally include a temperature range of 0-50°C and the use of a strong acid such as sulfuric acid or hydrochloric acid as the catalyst.
Industrial Production Methods
In an industrial setting, the production of this compound can be scaled up by using continuous flow reactors. These reactors allow for better control of reaction conditions, such as temperature and pressure, and can handle larger volumes of reactants. The use of a continuous flow reactor also improves the efficiency and yield of the reaction.
Chemical Reactions Analysis
Types of Reactions
4,4-Diethoxybut-1-ene can undergo various types of chemical reactions, including:
Oxidation: The compound can be oxidized to form 4,4-diethoxybutan-1-one using oxidizing agents such as potassium permanganate or chromium trioxide.
Reduction: Reduction of this compound can yield 4,4-diethoxybutane using reducing agents like lithium aluminum hydride.
Substitution: The ethoxy groups can be substituted with other functional groups through nucleophilic substitution reactions.
Common Reagents and Conditions
Oxidation: Potassium permanganate (KMnO4) in an acidic medium.
Reduction: Lithium aluminum hydride (LiAlH4) in anhydrous ether.
Substitution: Sodium ethoxide (NaOEt) in ethanol.
Major Products
Oxidation: 4,4-Diethoxybutan-1-one.
Reduction: 4,4-Diethoxybutane.
Substitution: Various substituted butenes depending on the nucleophile used.
Scientific Research Applications
4,4-Diethoxybut-1-ene has several applications in scientific research, including:
Chemistry: It is used as an intermediate in the synthesis of more complex organic molecules
Biology: The compound can be used in the study of enzyme-catalyzed reactions involving ether bonds.
Industry: this compound is used in the production of specialty chemicals and as a solvent in certain industrial processes.
Mechanism of Action
The mechanism by which 4,4-diethoxybut-1-ene exerts its effects depends on the specific reaction it undergoes For example, in oxidation reactions, the compound undergoes electron transfer processes that lead to the formation of oxidized products
Comparison with Similar Compounds
Similar Compounds
4,4-Dimethoxybut-1-ene: Similar in structure but with methoxy groups instead of ethoxy groups.
4,4-Diethoxybutan-1-ol: Contains a hydroxyl group in addition to the ethoxy groups.
4,4-Diethoxybutan-1-one: An oxidized form of 4,4-diethoxybut-1-ene.
Uniqueness
This compound is unique due to the presence of two ethoxy groups attached to the same carbon atom, which imparts distinct chemical properties and reactivity. This makes it a valuable intermediate in organic synthesis and a useful compound in various research applications.
Properties
IUPAC Name |
4,4-diethoxybut-1-ene | |
---|---|---|
Source | PubChem | |
URL | https://pubchem.ncbi.nlm.nih.gov | |
Description | Data deposited in or computed by PubChem | |
InChI |
InChI=1S/C8H16O2/c1-4-7-8(9-5-2)10-6-3/h4,8H,1,5-7H2,2-3H3 | |
Source | PubChem | |
URL | https://pubchem.ncbi.nlm.nih.gov | |
Description | Data deposited in or computed by PubChem | |
InChI Key |
PRCYIYOCMALJCX-UHFFFAOYSA-N | |
Source | PubChem | |
URL | https://pubchem.ncbi.nlm.nih.gov | |
Description | Data deposited in or computed by PubChem | |
Canonical SMILES |
CCOC(CC=C)OCC | |
Source | PubChem | |
URL | https://pubchem.ncbi.nlm.nih.gov | |
Description | Data deposited in or computed by PubChem | |
Molecular Formula |
C8H16O2 | |
Source | PubChem | |
URL | https://pubchem.ncbi.nlm.nih.gov | |
Description | Data deposited in or computed by PubChem | |
DSSTOX Substance ID |
DTXSID80292502 | |
Record name | 4,4-diethoxybut-1-ene | |
Source | EPA DSSTox | |
URL | https://comptox.epa.gov/dashboard/DTXSID80292502 | |
Description | DSSTox provides a high quality public chemistry resource for supporting improved predictive toxicology. | |
Molecular Weight |
144.21 g/mol | |
Source | PubChem | |
URL | https://pubchem.ncbi.nlm.nih.gov | |
Description | Data deposited in or computed by PubChem | |
CAS No. |
10602-36-5 | |
Record name | 10602-36-5 | |
Source | DTP/NCI | |
URL | https://dtp.cancer.gov/dtpstandard/servlet/dwindex?searchtype=NSC&outputformat=html&searchlist=83289 | |
Description | The NCI Development Therapeutics Program (DTP) provides services and resources to the academic and private-sector research communities worldwide to facilitate the discovery and development of new cancer therapeutic agents. | |
Explanation | Unless otherwise indicated, all text within NCI products is free of copyright and may be reused without our permission. Credit the National Cancer Institute as the source. | |
Record name | 4,4-diethoxybut-1-ene | |
Source | EPA DSSTox | |
URL | https://comptox.epa.gov/dashboard/DTXSID80292502 | |
Description | DSSTox provides a high quality public chemistry resource for supporting improved predictive toxicology. | |
Record name | 3-Butenal diethyl acetal | |
Source | European Chemicals Agency (ECHA) | |
URL | https://echa.europa.eu/information-on-chemicals | |
Description | The European Chemicals Agency (ECHA) is an agency of the European Union which is the driving force among regulatory authorities in implementing the EU's groundbreaking chemicals legislation for the benefit of human health and the environment as well as for innovation and competitiveness. | |
Explanation | Use of the information, documents and data from the ECHA website is subject to the terms and conditions of this Legal Notice, and subject to other binding limitations provided for under applicable law, the information, documents and data made available on the ECHA website may be reproduced, distributed and/or used, totally or in part, for non-commercial purposes provided that ECHA is acknowledged as the source: "Source: European Chemicals Agency, http://echa.europa.eu/". Such acknowledgement must be included in each copy of the material. ECHA permits and encourages organisations and individuals to create links to the ECHA website under the following cumulative conditions: Links can only be made to webpages that provide a link to the Legal Notice page. | |
Retrosynthesis Analysis
AI-Powered Synthesis Planning: Our tool employs the Template_relevance Pistachio, Template_relevance Bkms_metabolic, Template_relevance Pistachio_ringbreaker, Template_relevance Reaxys, Template_relevance Reaxys_biocatalysis model, leveraging a vast database of chemical reactions to predict feasible synthetic routes.
One-Step Synthesis Focus: Specifically designed for one-step synthesis, it provides concise and direct routes for your target compounds, streamlining the synthesis process.
Accurate Predictions: Utilizing the extensive PISTACHIO, BKMS_METABOLIC, PISTACHIO_RINGBREAKER, REAXYS, REAXYS_BIOCATALYSIS database, our tool offers high-accuracy predictions, reflecting the latest in chemical research and data.
Strategy Settings
Precursor scoring | Relevance Heuristic |
---|---|
Min. plausibility | 0.01 |
Model | Template_relevance |
Template Set | Pistachio/Bkms_metabolic/Pistachio_ringbreaker/Reaxys/Reaxys_biocatalysis |
Top-N result to add to graph | 6 |
Feasible Synthetic Routes
Q1: What makes 4,4-diethoxybut-1-ene a useful reagent in organic synthesis?
A1: this compound serves as a valuable precursor for synthesizing homoallyl and homocinnamyl skeletons. [] This compound readily participates in reactions with various electrophiles, introducing a four-carbon chain with a versatile terminal alkene. This alkene can be further functionalized, broadening the synthetic possibilities. For instance, the research by Hosomi et al. [] demonstrated its utility in reactions with aldehydes mediated by Lewis acids, leading to the formation of homoallylic alcohols.
Q2: Are there any examples of diastereoselective reactions involving this compound derivatives?
A2: While the provided abstracts don't specifically mention this compound, they highlight the diastereoselective allylation of α-hydroxy Schiff bases using functionalized allyl bromides. [] Although structurally different, this research demonstrates the possibility of achieving diastereoselectivity in reactions with similar allylic reagents, suggesting potential avenues for exploring diastereoselective transformations involving this compound or its derivatives. Further research could investigate if similar selectivity can be achieved by modifying the reaction conditions or employing chiral catalysts in conjunction with this compound.
Disclaimer and Information on In-Vitro Research Products
Please be aware that all articles and product information presented on BenchChem are intended solely for informational purposes. The products available for purchase on BenchChem are specifically designed for in-vitro studies, which are conducted outside of living organisms. In-vitro studies, derived from the Latin term "in glass," involve experiments performed in controlled laboratory settings using cells or tissues. It is important to note that these products are not categorized as medicines or drugs, and they have not received approval from the FDA for the prevention, treatment, or cure of any medical condition, ailment, or disease. We must emphasize that any form of bodily introduction of these products into humans or animals is strictly prohibited by law. It is essential to adhere to these guidelines to ensure compliance with legal and ethical standards in research and experimentation.