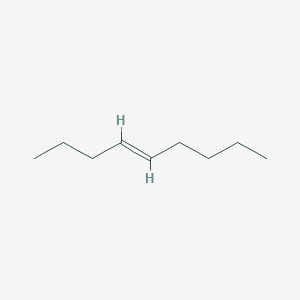
Trans-4-NONENE
Overview
Description
Trans-4-Nonene: is an unsaturated hydrocarbon with the molecular formula C₉H₁₈ . It is a colorless liquid with a distinct odor and is one of the isomers of nonene. The compound is characterized by the presence of a double bond between the fourth and fifth carbon atoms in the trans configuration, which means the hydrogen atoms attached to the double-bonded carbons are on opposite sides.
Preparation Methods
Synthetic Routes and Reaction Conditions: Trans-4-Nonene can be synthesized through various methods, including:
Alkylation Reactions: One common method involves the alkylation of butenes with pentenes in the presence of a catalyst.
Dehydration of Alcohols: Another method is the dehydration of 4-nonanol using an acid catalyst, such as sulfuric acid, to yield this compound.
Industrial Production Methods: In industrial settings, this compound is often produced through the oligomerization of ethylene. This process involves the polymerization of ethylene in the presence of a catalyst to form higher alkenes, including nonenes. The mixture is then separated and purified to obtain this compound.
Chemical Reactions Analysis
Types of Reactions:
Oxidation: Trans-4-Nonene can undergo oxidation reactions to form various oxygenated compounds, such as alcohols, aldehydes, and carboxylic acids.
Reduction: It can be reduced to form saturated hydrocarbons, such as nonane, using hydrogenation reactions with catalysts like palladium or platinum.
Substitution: this compound can participate in substitution reactions, where the double bond reacts with halogens or other electrophiles to form halogenated products.
Common Reagents and Conditions:
Oxidation: Common oxidizing agents include potassium permanganate and ozone.
Reduction: Hydrogen gas in the presence of a palladium or platinum catalyst.
Substitution: Halogens like chlorine or bromine in the presence of light or a catalyst.
Major Products Formed:
Oxidation: 4-Nonanol, 4-Nonanone, and nonanoic acid.
Reduction: Nonane.
Substitution: 4-Chlorononene, 4-Bromononene.
Scientific Research Applications
Trans-4-Nonene has several applications in scientific research and industry:
Chemistry: It is used as an intermediate in the synthesis of various organic compounds, including polymers, solvents, and surfactants.
Biology: this compound is studied for its potential biological activities and interactions with enzymes and other biomolecules.
Medicine: Research is ongoing to explore its potential use in pharmaceuticals and as a precursor for drug synthesis.
Industry: It is used in the production of plasticizers, lubricants, and other industrial chemicals.
Mechanism of Action
The mechanism of action of trans-4-Nonene involves its interactions with various molecular targets and pathways. As an unsaturated hydrocarbon, it can participate in addition reactions with electrophiles, leading to the formation of new compounds. Its double bond allows it to act as a nucleophile, reacting with electrophilic species in various chemical processes.
Comparison with Similar Compounds
Cis-4-Nonene: The cis isomer of 4-Nonene, where the hydrogen atoms on the double-bonded carbons are on the same side.
1-Nonene: An isomer with the double bond at the first carbon position.
2-Nonene: An isomer with the double bond at the second carbon position.
Trans-4-Nonene is unique due to its trans configuration, which affects its physical properties, such as boiling point and density, as well as its reactivity in chemical reactions.
Biological Activity
Trans-4-nonene is an unsaturated hydrocarbon with the molecular formula and a CAS number of 10405-85-3. It has garnered attention in recent years for its potential biological activities, particularly in relation to its interactions with various biomolecules and its role in metabolic processes. This article provides a comprehensive overview of the biological activity of this compound, including research findings, mechanisms of action, and relevant case studies.
This compound is characterized by its trans configuration , which influences its chemical reactivity. The presence of a double bond allows this compound to act as a nucleophile, participating in addition reactions with electrophiles. This property is significant in biochemical contexts, where this compound can interact with various enzymes and cellular components.
Key Mechanisms:
- Electrophilic Addition : this compound can undergo reactions with electrophilic species, leading to the formation of new compounds that may exhibit biological activity.
- Metabolic Pathways : The compound is involved in lipid peroxidation processes, where it can be metabolized into other biologically active aldehydes such as 4-hydroxy-2-nonenal (HNE) .
Biological Activities
Research indicates that this compound may exert several biological effects:
- Antimicrobial Activity : Studies have shown that certain aldehydes derived from lipid peroxidation, including HNE, can influence bacterial survival and growth. For instance, exposure to HNE has been linked to delayed growth in Listeria monocytogenes, suggesting that similar effects could be expected from this compound due to its structural similarity .
- Cytotoxic Effects : this compound may induce cytotoxicity through the formation of protein adducts, which can disrupt cellular functions. The degree of toxicity is often dependent on concentration and exposure duration .
- Signaling Molecule : As a product of lipid peroxidation, this compound might play a role in redox signaling pathways. Its derivatives have been implicated in modulating cellular responses to oxidative stress .
Table 1: Summary of Biological Activities Associated with this compound
Case Study: Antimicrobial Properties
A study investigating the effects of HNE on Listeria monocytogenes demonstrated that while HNE exhibited limited bactericidal activity, it significantly delayed bacterial growth at higher concentrations. This suggests that this compound could similarly influence bacterial physiology by affecting metabolic pathways and inducing stress responses .
Properties
IUPAC Name |
(E)-non-4-ene | |
---|---|---|
Source | PubChem | |
URL | https://pubchem.ncbi.nlm.nih.gov | |
Description | Data deposited in or computed by PubChem | |
InChI |
InChI=1S/C9H18/c1-3-5-7-9-8-6-4-2/h7,9H,3-6,8H2,1-2H3/b9-7+ | |
Source | PubChem | |
URL | https://pubchem.ncbi.nlm.nih.gov | |
Description | Data deposited in or computed by PubChem | |
InChI Key |
KPADFPAILITQBG-VQHVLOKHSA-N | |
Source | PubChem | |
URL | https://pubchem.ncbi.nlm.nih.gov | |
Description | Data deposited in or computed by PubChem | |
Canonical SMILES |
CCCCC=CCCC | |
Source | PubChem | |
URL | https://pubchem.ncbi.nlm.nih.gov | |
Description | Data deposited in or computed by PubChem | |
Isomeric SMILES |
CCCC/C=C/CCC | |
Source | PubChem | |
URL | https://pubchem.ncbi.nlm.nih.gov | |
Description | Data deposited in or computed by PubChem | |
Molecular Formula |
C9H18 | |
Source | PubChem | |
URL | https://pubchem.ncbi.nlm.nih.gov | |
Description | Data deposited in or computed by PubChem | |
DSSTOX Substance ID |
DTXSID60873999 | |
Record name | (4E)-4-Nonene | |
Source | EPA DSSTox | |
URL | https://comptox.epa.gov/dashboard/DTXSID60873999 | |
Description | DSSTox provides a high quality public chemistry resource for supporting improved predictive toxicology. | |
Molecular Weight |
126.24 g/mol | |
Source | PubChem | |
URL | https://pubchem.ncbi.nlm.nih.gov | |
Description | Data deposited in or computed by PubChem | |
CAS No. |
10405-85-3 | |
Record name | 4-Nonene, (4E)- | |
Source | ChemIDplus | |
URL | https://pubchem.ncbi.nlm.nih.gov/substance/?source=chemidplus&sourceid=0010405853 | |
Description | ChemIDplus is a free, web search system that provides access to the structure and nomenclature authority files used for the identification of chemical substances cited in National Library of Medicine (NLM) databases, including the TOXNET system. | |
Record name | (4E)-4-Nonene | |
Source | EPA DSSTox | |
URL | https://comptox.epa.gov/dashboard/DTXSID60873999 | |
Description | DSSTox provides a high quality public chemistry resource for supporting improved predictive toxicology. | |
Record name | 4-NONENE, (4E)- | |
Source | FDA Global Substance Registration System (GSRS) | |
URL | https://gsrs.ncats.nih.gov/ginas/app/beta/substances/U5OC0PYC75 | |
Description | The FDA Global Substance Registration System (GSRS) enables the efficient and accurate exchange of information on what substances are in regulated products. Instead of relying on names, which vary across regulatory domains, countries, and regions, the GSRS knowledge base makes it possible for substances to be defined by standardized, scientific descriptions. | |
Explanation | Unless otherwise noted, the contents of the FDA website (www.fda.gov), both text and graphics, are not copyrighted. They are in the public domain and may be republished, reprinted and otherwise used freely by anyone without the need to obtain permission from FDA. Credit to the U.S. Food and Drug Administration as the source is appreciated but not required. | |
Retrosynthesis Analysis
AI-Powered Synthesis Planning: Our tool employs the Template_relevance Pistachio, Template_relevance Bkms_metabolic, Template_relevance Pistachio_ringbreaker, Template_relevance Reaxys, Template_relevance Reaxys_biocatalysis model, leveraging a vast database of chemical reactions to predict feasible synthetic routes.
One-Step Synthesis Focus: Specifically designed for one-step synthesis, it provides concise and direct routes for your target compounds, streamlining the synthesis process.
Accurate Predictions: Utilizing the extensive PISTACHIO, BKMS_METABOLIC, PISTACHIO_RINGBREAKER, REAXYS, REAXYS_BIOCATALYSIS database, our tool offers high-accuracy predictions, reflecting the latest in chemical research and data.
Strategy Settings
Precursor scoring | Relevance Heuristic |
---|---|
Min. plausibility | 0.01 |
Model | Template_relevance |
Template Set | Pistachio/Bkms_metabolic/Pistachio_ringbreaker/Reaxys/Reaxys_biocatalysis |
Top-N result to add to graph | 6 |
Feasible Synthetic Routes
Q1: How does the structure of trans-4-nonene influence its reactivity in the presence of the platinum-zeolite catalyst modified with organosilicon alkoxide?
A1: The research paper focuses on the regioselective hydrogenation of unsaturated compounds, specifically comparing the reaction rates of 1-nonene (terminal alkene) and this compound (internal alkene) []. The study demonstrates that the platinum-zeolite catalyst modified with diphenyldiethoxysilane exhibits a preference for hydrogenating terminal carbon-carbon double bonds over internal ones []. This suggests that the steric hindrance around the double bond in this compound hinders its interaction with the catalytic sites compared to the more accessible terminal double bond in 1-nonene.
Disclaimer and Information on In-Vitro Research Products
Please be aware that all articles and product information presented on BenchChem are intended solely for informational purposes. The products available for purchase on BenchChem are specifically designed for in-vitro studies, which are conducted outside of living organisms. In-vitro studies, derived from the Latin term "in glass," involve experiments performed in controlled laboratory settings using cells or tissues. It is important to note that these products are not categorized as medicines or drugs, and they have not received approval from the FDA for the prevention, treatment, or cure of any medical condition, ailment, or disease. We must emphasize that any form of bodily introduction of these products into humans or animals is strictly prohibited by law. It is essential to adhere to these guidelines to ensure compliance with legal and ethical standards in research and experimentation.