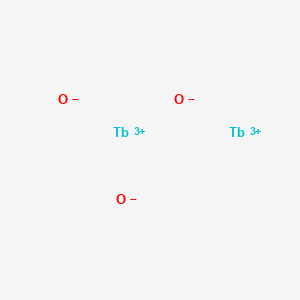
Terbium oxide (Tb2O3)
Overview
Description
Mechanism of Action
Target of Action
Terbium oxide primarily targets protons , conducting them in a manner that is enhanced when doped with calcium . This property makes it a p-type semiconductor .
Mode of Action
Terbium oxide interacts with its targets (protons) by conducting them. This interaction is enhanced when the compound is doped with calcium, which increases its conductivity . The compound can be prepared by the reduction of Tb4O7 in hydrogen at 1300 °C for 24 hours .
Biochemical Pathways
It is known that the compound can work like v2o5 as a redox catalyst in reactions involving oxygen . This suggests that it may play a role in redox reactions and related biochemical pathways.
Pharmacokinetics
It is known that the compound is a basic oxide and easily dissolved to dilute acids . This could potentially influence its bioavailability.
Result of Action
The result of terbium oxide’s action is the formation of almost colorless terbium salt when it is dissolved in dilute acids . This reaction is represented by the equation: Tb2O3 + 6H+ → 2Tb3+ + 3H2O .
Action Environment
The action of terbium oxide can be influenced by environmental factors such as temperature. For example, the compound loses O2 when heated at high temperatures . At more moderate temperatures (around 350 °C), it reversibly loses oxygen . These properties allow it to function as a redox catalyst in reactions involving oxygen .
Biochemical Analysis
Biochemical Properties
Terbium oxide (Tb2O3) plays a significant role in biochemical reactions, particularly in the field of electrochemical sensing. It has been investigated for its non-enzymatic electrochemical detection capabilities, especially for hydrogen peroxide (H2O2) detection . The interaction between terbium oxide and hydrogen peroxide involves the catalytic reduction of H2O2, which is facilitated by the high surface area and catalytic activity of Tb2O3 nanotubes. This interaction is crucial for developing sensitive and selective biosensors for detecting reactive oxygen species in biological systems.
Cellular Effects
The effects of terbium oxide (Tb2O3) on various types of cells and cellular processes have been studied to understand its potential biomedical applications. Terbium oxide nanoparticles have been shown to exhibit biocompatibility and low cytotoxicity, making them suitable for use in biological systems . In cellular studies, terbium oxide has been observed to influence cell function by modulating cell signaling pathways, gene expression, and cellular metabolism. For instance, terbium oxide nanoparticles can induce oxidative stress in cells, leading to changes in the expression of antioxidant enzymes and other stress-related genes.
Molecular Mechanism
At the molecular level, terbium oxide (Tb2O3) exerts its effects through various mechanisms. One of the primary mechanisms involves the interaction of terbium ions (Tb3+) with biomolecules, such as proteins and enzymes. Terbium ions can bind to specific sites on proteins, altering their conformation and activity. Additionally, terbium oxide can act as an enzyme inhibitor or activator, depending on the context of the biochemical reaction . These interactions can lead to changes in gene expression and cellular responses, highlighting the importance of understanding the molecular mechanisms of terbium oxide in biological systems.
Temporal Effects in Laboratory Settings
The stability and degradation of terbium oxide (Tb2O3) in laboratory settings are critical factors that influence its long-term effects on cellular function. Studies have shown that terbium oxide maintains its structural integrity and catalytic activity over extended periods under controlled conditions . The degradation of terbium oxide can occur in the presence of strong acids or high temperatures, leading to the formation of terbium salts. Long-term exposure to terbium oxide in in vitro and in vivo studies has demonstrated its potential to induce chronic oxidative stress and alter cellular homeostasis.
Dosage Effects in Animal Models
The effects of terbium oxide (Tb2O3) vary with different dosages in animal models. Low doses of terbium oxide have been found to be non-toxic and well-tolerated, while high doses can lead to adverse effects, such as oxidative damage and inflammation . Threshold effects have been observed, where specific concentrations of terbium oxide induce significant biological responses. Understanding the dosage-dependent effects of terbium oxide is essential for its safe and effective use in biomedical applications.
Metabolic Pathways
Terbium oxide (Tb2O3) is involved in various metabolic pathways, interacting with enzymes and cofactors that regulate cellular metabolism. The presence of terbium ions can influence metabolic flux and alter metabolite levels in cells. For example, terbium oxide can affect the activity of enzymes involved in oxidative phosphorylation and glycolysis, leading to changes in cellular energy production . These interactions highlight the potential of terbium oxide to modulate metabolic pathways and impact cellular function.
Transport and Distribution
The transport and distribution of terbium oxide (Tb2O3) within cells and tissues are mediated by specific transporters and binding proteins. Terbium ions can be transported across cell membranes through ion channels and transporters, facilitating their accumulation in specific cellular compartments . The distribution of terbium oxide within tissues is influenced by factors such as particle size, surface charge, and the presence of targeting ligands. Understanding the transport and distribution mechanisms of terbium oxide is crucial for optimizing its biomedical applications.
Subcellular Localization
The subcellular localization of terbium oxide (Tb2O3) plays a significant role in its activity and function. Terbium oxide nanoparticles can be directed to specific cellular compartments, such as the mitochondria, nucleus, or lysosomes, through targeting signals or post-translational modifications . The localization of terbium oxide within these compartments can influence its interactions with biomolecules and its overall biological effects. For instance, the accumulation of terbium oxide in the mitochondria can impact mitochondrial function and induce oxidative stress.
Preparation Methods
Synthetic Routes and Reaction Conditions
Terbium oxide can be prepared by the reduction of terbium(IV) oxide (Tb₄O₇) in a hydrogen atmosphere at 1300°C for 24 hours . The reaction is as follows: [ \text{Tb}_4\text{O}_7 + \text{H}_2 \rightarrow 2 \text{Tb}_2\text{O}_3 + \text{H}_2\text{O} ]
Another method involves the calcination of terbium hydroxide (Tb(OH)₃) nanotubes prepared by a template-assisted hydrothermal route . The oxide nanotubes have outer diameters ranging from 80 to 100 nm, wall thickness of about 30 nm, and lengths up to several micrometers .
Industrial Production Methods
In industrial settings, terbium oxide is often produced by heating the metal oxalate. This method is preferred due to its efficiency and the quality of the resulting terbium oxide .
Chemical Reactions Analysis
Types of Reactions
Terbium oxide undergoes several types of chemical reactions, including:
Reduction: As mentioned earlier, terbium(IV) oxide can be reduced to terbium oxide using hydrogen.
Acid-Base Reactions: Terbium oxide is a basic oxide and easily dissolves in dilute acids to form almost colorless terbium salts. The reaction with hydrochloric acid is as follows: [ \text{Tb}_2\text{O}_3 + 6 \text{H}^+ \rightarrow 2 \text{Tb}^{3+} + 3 \text{H}_2\text{O} ]
Common Reagents and Conditions
Common reagents used in reactions with terbium oxide include hydrogen for reduction and various acids for dissolution and salt formation .
Major Products Formed
The major products formed from these reactions include terbium salts such as terbium chloride when reacted with hydrochloric acid .
Scientific Research Applications
Terbium oxide has a wide range of scientific research applications:
Magneto-Optical Ceramics: Terbium oxide is used in the development of magneto-optical transparent ceramics.
Glass, Optic, and Ceramic Applications: Due to its thermal stability and insolubility, terbium oxide is used in the production of glass, optic, and ceramic materials.
Comparison with Similar Compounds
Terbium oxide can be compared with other rare earth oxides such as gadolinium oxide (Gd₂O₃) and dysprosium oxide (Dy₂O₃). While all these compounds have similar crystal structures and are used in various high-tech applications, terbium oxide is unique due to its higher Verdet constant and superior magneto-optical properties . This makes it particularly valuable in applications requiring high magnetic sensitivity and optical clarity .
List of Similar Compounds
- Gadolinium oxide (Gd₂O₃)
- Dysprosium oxide (Dy₂O₃)
- Holmium oxide (Ho₂O₃)
- Praseodymium oxide (Pr₆O₁₁)
Properties
IUPAC Name |
oxygen(2-);terbium(3+) | |
---|---|---|
Source | PubChem | |
URL | https://pubchem.ncbi.nlm.nih.gov | |
Description | Data deposited in or computed by PubChem | |
InChI |
InChI=1S/3O.2Tb/q3*-2;2*+3 | |
Source | PubChem | |
URL | https://pubchem.ncbi.nlm.nih.gov | |
Description | Data deposited in or computed by PubChem | |
InChI Key |
SCRZPWWVSXWCMC-UHFFFAOYSA-N | |
Source | PubChem | |
URL | https://pubchem.ncbi.nlm.nih.gov | |
Description | Data deposited in or computed by PubChem | |
Canonical SMILES |
[O-2].[O-2].[O-2].[Tb+3].[Tb+3] | |
Source | PubChem | |
URL | https://pubchem.ncbi.nlm.nih.gov | |
Description | Data deposited in or computed by PubChem | |
Molecular Formula |
O3Tb2 | |
Source | PubChem | |
URL | https://pubchem.ncbi.nlm.nih.gov | |
Description | Data deposited in or computed by PubChem | |
DSSTOX Substance ID |
DTXSID401014252 | |
Record name | Terbium oxide (Tb2O3) | |
Source | EPA DSSTox | |
URL | https://comptox.epa.gov/dashboard/DTXSID401014252 | |
Description | DSSTox provides a high quality public chemistry resource for supporting improved predictive toxicology. | |
Molecular Weight |
365.849 g/mol | |
Source | PubChem | |
URL | https://pubchem.ncbi.nlm.nih.gov | |
Description | Data deposited in or computed by PubChem | |
CAS No. |
12036-41-8, 12037-01-3 | |
Record name | Terbium oxide (Tb2O3) | |
Source | ChemIDplus | |
URL | https://pubchem.ncbi.nlm.nih.gov/substance/?source=chemidplus&sourceid=0012036418 | |
Description | ChemIDplus is a free, web search system that provides access to the structure and nomenclature authority files used for the identification of chemical substances cited in National Library of Medicine (NLM) databases, including the TOXNET system. | |
Record name | Terbium oxide (Tb4O7) | |
Source | ChemIDplus | |
URL | https://pubchem.ncbi.nlm.nih.gov/substance/?source=chemidplus&sourceid=0012037013 | |
Description | ChemIDplus is a free, web search system that provides access to the structure and nomenclature authority files used for the identification of chemical substances cited in National Library of Medicine (NLM) databases, including the TOXNET system. | |
Record name | Terbium oxide (Tb2O3) | |
Source | EPA Chemicals under the TSCA | |
URL | https://www.epa.gov/chemicals-under-tsca | |
Description | EPA Chemicals under the Toxic Substances Control Act (TSCA) collection contains information on chemicals and their regulations under TSCA, including non-confidential content from the TSCA Chemical Substance Inventory and Chemical Data Reporting. | |
Record name | Terbium oxide (Tb2O3) | |
Source | EPA DSSTox | |
URL | https://comptox.epa.gov/dashboard/DTXSID401014252 | |
Description | DSSTox provides a high quality public chemistry resource for supporting improved predictive toxicology. | |
Q1: What makes Terbium oxide interesting for magneto-optical applications?
A: Terbium oxide nanoparticles, along with terbium fluoride and terbium oxyfluoride, exhibit intriguing magneto-optical properties. [] This means they interact with both magnetic and light fields, making them potentially valuable for applications like optical sensors and magnetic storage devices.
Q2: How does the synthesis method affect the luminescent properties of Terbium oxide?
A: Research shows that Terbium oxide particles synthesized via precipitation using ammonium carbonate display a strong cyan luminescence. [] The intensity of this luminescence, particularly the peak at 493 nm (attributed to the 5D4-7F6 transition), is influenced by the precursor molarity used during synthesis. Higher molarity leads to a shift towards the blue region in the CIE diagram, indicating a change in the perceived color. []
Q3: Are there any challenges in utilizing the luminescence of Terbium oxide for applications like high-resolution imaging?
A: The long decay time of Tb(3+) luminescence in materials like silicate glass can lead to "afterglow," a secondary, long-lived component in the luminescence decay curve. [] This afterglow can blur images of transient events. Understanding the factors influencing this afterglow is crucial for optimizing Terbium oxide's use in applications requiring high temporal resolution. []
Q4: What other applications does Terbium oxide have in electronics?
A: Research indicates that Terbium oxide exhibits a high dielectric constant, making it a potential candidate for use as a dielectric material in electronic devices. [] This property has been specifically studied in the context of strained-Si:C substrates, highlighting its potential for advanced electronic applications. []
Disclaimer and Information on In-Vitro Research Products
Please be aware that all articles and product information presented on BenchChem are intended solely for informational purposes. The products available for purchase on BenchChem are specifically designed for in-vitro studies, which are conducted outside of living organisms. In-vitro studies, derived from the Latin term "in glass," involve experiments performed in controlled laboratory settings using cells or tissues. It is important to note that these products are not categorized as medicines or drugs, and they have not received approval from the FDA for the prevention, treatment, or cure of any medical condition, ailment, or disease. We must emphasize that any form of bodily introduction of these products into humans or animals is strictly prohibited by law. It is essential to adhere to these guidelines to ensure compliance with legal and ethical standards in research and experimentation.