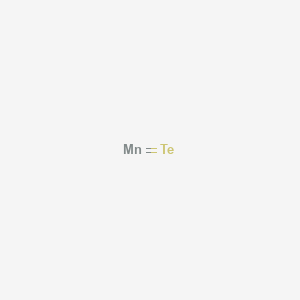
Manganese telluride
Overview
Description
Manganese telluride is an inorganic compound with the chemical formula MnTe. It is known for its unique magnetic properties, having been recently classified as an altermagnetic material. This compound has a hexagonal crystal structure and is insoluble in water. This compound has a Néel temperature of 307 K, indicating its antiferromagnetic to paramagnetic transition temperature .
Mechanism of Action
Target of Action
Manganese telluride (MnTe) is primarily used in the field of energy storage and conversion, specifically in supercapacitor applications . It has also been identified as a potential thermoelectric material . The primary targets of MnTe are the electrochemical systems where it acts as an effective material for energy storage .
Mode of Action
This compound interacts with its targets by facilitating electron transfer and augmenting the active electrochemical surface area . This is achieved through the effective incorporation of MnTe into graphene oxide sheets, forming a MnTe/GO nanostructure . This interaction results in enhanced electrochemical capabilities .
Biochemical Pathways
Its action involves the electron transfer processes in electrochemical systems .
Pharmacokinetics
For instance, the MnTe/GO nanostructure exhibits a heightened specific capacity (Csp) of 2203 F g −1 at a current density of 2 A g −1 .
Result of Action
The result of this compound’s action is the enhancement of the performance of energy storage systems. Specifically, the MnTe/GO nanostructure demonstrates an impressive retention rate of 99% over 2000 cycles, highlighting its superior stability . This makes it a promising candidate for next-generation, high-performance supercapacitor applications .
Action Environment
The action of this compound can be influenced by environmental factors. For instance, the synthesis of MnTe/GO nanostructure involves a hydrothermal process . .
Preparation Methods
Synthetic Routes and Reaction Conditions: Manganese telluride can be synthesized through the fusion of manganese and tellurium in a vacuum. This method involves heating the elements together until they react to form the compound .
Industrial Production Methods: In industrial settings, this compound can be produced using hydrothermal synthesis. This method involves dissolving manganese chloride dihydrate and tellurium powder in a potassium hydroxide solution, followed by heating the mixture under high pressure . Another method includes chemical vapor deposition, where manganese and tellurium precursors are vaporized and deposited onto a substrate to form thin films of this compound .
Chemical Reactions Analysis
Types of Reactions: Manganese telluride undergoes various chemical reactions, including oxidation, reduction, and substitution reactions.
Common Reagents and Conditions:
Oxidation: this compound can be oxidized using oxygen or other oxidizing agents, leading to the formation of manganese oxides and tellurium oxides.
Reduction: Reduction reactions can be carried out using hydrogen or other reducing agents to convert this compound back to its elemental forms.
Substitution: Substitution reactions involve replacing tellurium with other chalcogens like sulfur or selenium under specific conditions.
Major Products Formed: The major products formed from these reactions include manganese oxides, tellurium oxides, and substituted manganese chalcogenides .
Scientific Research Applications
Manganese telluride has a wide range of scientific research applications:
Biology and Medicine: While direct applications in biology and medicine are limited, this compound’s magnetic properties make it a candidate for research in magnetic resonance imaging (MRI) contrast agents.
Industry: this compound is used in the development of high-performance supercapacitors and lithium-ion batteries due to its excellent electrochemical properties.
Comparison with Similar Compounds
- Manganese oxide (MnO)
- Manganese sulfide (MnS)
- Manganese selenide (MnSe)
- Manganese ditelluride (MnTe2)
Comparison: Manganese telluride is unique among these compounds due to its altermagnetic properties, which distinguish it from the more common antiferromagnetic or ferromagnetic materials. Unlike manganese oxide, sulfide, and selenide, this compound exhibits a Néel temperature of 307 K and specific spin splitting characteristics that make it particularly valuable for advanced magnetic applications .
Properties
IUPAC Name |
tellanylidenemanganese | |
---|---|---|
Source | PubChem | |
URL | https://pubchem.ncbi.nlm.nih.gov | |
Description | Data deposited in or computed by PubChem | |
InChI |
InChI=1S/Mn.Te | |
Source | PubChem | |
URL | https://pubchem.ncbi.nlm.nih.gov | |
Description | Data deposited in or computed by PubChem | |
InChI Key |
VMINMXIEZOMBRH-UHFFFAOYSA-N | |
Source | PubChem | |
URL | https://pubchem.ncbi.nlm.nih.gov | |
Description | Data deposited in or computed by PubChem | |
Canonical SMILES |
[Mn]=[Te] | |
Source | PubChem | |
URL | https://pubchem.ncbi.nlm.nih.gov | |
Description | Data deposited in or computed by PubChem | |
Molecular Formula |
MnTe | |
Record name | manganese(II) telluride | |
Source | Wikipedia | |
URL | https://en.wikipedia.org/wiki/Manganese(II)_telluride | |
Description | Chemical information link to Wikipedia. | |
Source | PubChem | |
URL | https://pubchem.ncbi.nlm.nih.gov | |
Description | Data deposited in or computed by PubChem | |
DSSTOX Substance ID |
DTXSID001014212 | |
Record name | Manganese(II) telluride | |
Source | EPA DSSTox | |
URL | https://comptox.epa.gov/dashboard/DTXSID001014212 | |
Description | DSSTox provides a high quality public chemistry resource for supporting improved predictive toxicology. | |
Molecular Weight |
182.5 g/mol | |
Source | PubChem | |
URL | https://pubchem.ncbi.nlm.nih.gov | |
Description | Data deposited in or computed by PubChem | |
CAS No. |
12032-88-1 | |
Record name | Manganese telluride (MnTe) | |
Source | CAS Common Chemistry | |
URL | https://commonchemistry.cas.org/detail?cas_rn=12032-88-1 | |
Description | CAS Common Chemistry is an open community resource for accessing chemical information. Nearly 500,000 chemical substances from CAS REGISTRY cover areas of community interest, including common and frequently regulated chemicals, and those relevant to high school and undergraduate chemistry classes. This chemical information, curated by our expert scientists, is provided in alignment with our mission as a division of the American Chemical Society. | |
Explanation | The data from CAS Common Chemistry is provided under a CC-BY-NC 4.0 license, unless otherwise stated. | |
Record name | Manganese telluride (MnTe) | |
Source | ChemIDplus | |
URL | https://pubchem.ncbi.nlm.nih.gov/substance/?source=chemidplus&sourceid=0012032881 | |
Description | ChemIDplus is a free, web search system that provides access to the structure and nomenclature authority files used for the identification of chemical substances cited in National Library of Medicine (NLM) databases, including the TOXNET system. | |
Record name | Manganese telluride (MnTe) | |
Source | EPA Chemicals under the TSCA | |
URL | https://www.epa.gov/chemicals-under-tsca | |
Description | EPA Chemicals under the Toxic Substances Control Act (TSCA) collection contains information on chemicals and their regulations under TSCA, including non-confidential content from the TSCA Chemical Substance Inventory and Chemical Data Reporting. | |
Record name | Manganese(II) telluride | |
Source | EPA DSSTox | |
URL | https://comptox.epa.gov/dashboard/DTXSID001014212 | |
Description | DSSTox provides a high quality public chemistry resource for supporting improved predictive toxicology. | |
Record name | Manganese telluride | |
Source | European Chemicals Agency (ECHA) | |
URL | https://echa.europa.eu/substance-information/-/substanceinfo/100.031.607 | |
Description | The European Chemicals Agency (ECHA) is an agency of the European Union which is the driving force among regulatory authorities in implementing the EU's groundbreaking chemicals legislation for the benefit of human health and the environment as well as for innovation and competitiveness. | |
Explanation | Use of the information, documents and data from the ECHA website is subject to the terms and conditions of this Legal Notice, and subject to other binding limitations provided for under applicable law, the information, documents and data made available on the ECHA website may be reproduced, distributed and/or used, totally or in part, for non-commercial purposes provided that ECHA is acknowledged as the source: "Source: European Chemicals Agency, http://echa.europa.eu/". Such acknowledgement must be included in each copy of the material. ECHA permits and encourages organisations and individuals to create links to the ECHA website under the following cumulative conditions: Links can only be made to webpages that provide a link to the Legal Notice page. | |
Disclaimer and Information on In-Vitro Research Products
Please be aware that all articles and product information presented on BenchChem are intended solely for informational purposes. The products available for purchase on BenchChem are specifically designed for in-vitro studies, which are conducted outside of living organisms. In-vitro studies, derived from the Latin term "in glass," involve experiments performed in controlled laboratory settings using cells or tissues. It is important to note that these products are not categorized as medicines or drugs, and they have not received approval from the FDA for the prevention, treatment, or cure of any medical condition, ailment, or disease. We must emphasize that any form of bodily introduction of these products into humans or animals is strictly prohibited by law. It is essential to adhere to these guidelines to ensure compliance with legal and ethical standards in research and experimentation.