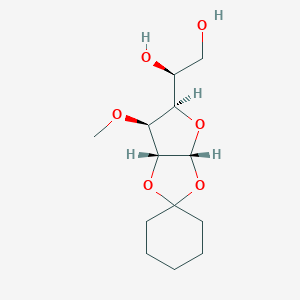
1,2-O-Cyclohexylidene-3-O-methyl-alpha-D-glucofuranose
Overview
Description
1,2-O-Cyclohexylidene-3-O-methyl-α-D-glucofuranose (CAS 13322-87-7) is a chemically modified derivative of α-D-glucofuranose, featuring a cyclohexylidene group at the 1,2-positions and a methyl ether at the 3-O position. Its molecular formula is C₁₃H₂₂O₆, with a molecular weight of 274.31 g/mol . The cyclohexylidene group acts as a protective moiety, selectively removable under acidic conditions, while the 3-O-methyl group enhances stability against oxidation and enzymatic degradation . This compound is widely employed in synthetic carbohydrate chemistry as a key intermediate for constructing oligosaccharides, glycoconjugates, and glycomimetics. Its solubility in organic solvents (e.g., DCM, DMF) and compatibility with glycosylation reactions make it indispensable for studying enzyme kinetics and carbohydrate-protein interactions .
Preparation Methods
Synthetic Routes and Reaction Conditions
1,2-O-Cyclohexylidene-3-O-methyl-alpha-D-glucofuranose can be synthesized through the cyclization of glucofuranose derivatives. The reaction typically involves the use of cyclohexanone and methanol in the presence of an acid catalyst. The reaction conditions often include:
Temperature: Room temperature to 50°C
Catalyst: Acidic catalysts such as hydrochloric acid or sulfuric acid
Solvent: Methanol or other suitable organic solvents
Industrial Production Methods
Industrial production of this compound follows similar synthetic routes but on a larger scale. The process involves:
Reactants: Glucofuranose derivatives, cyclohexanone, and methanol
Catalysts: Acidic catalysts
Reaction Conditions: Controlled temperature and pressure to optimize yield and purity
Chemical Reactions Analysis
Types of Reactions
1,2-O-Cyclohexylidene-3-O-methyl-alpha-D-glucofuranose undergoes various chemical reactions, including:
Oxidation: The compound can be oxidized to form corresponding carboxylic acids or ketones.
Reduction: Reduction reactions can convert the compound into alcohols or other reduced forms.
Substitution: The compound can undergo substitution reactions where functional groups are replaced by other groups.
Common Reagents and Conditions
Oxidation: Reagents such as potassium permanganate or chromium trioxide in acidic or basic conditions.
Reduction: Reagents like sodium borohydride or lithium aluminum hydride.
Substitution: Reagents such as halogens or nucleophiles in the presence of catalysts.
Major Products Formed
Oxidation: Carboxylic acids, ketones
Reduction: Alcohols
Substitution: Various substituted derivatives depending on the reagents used
Scientific Research Applications
1,2-O-Cyclohexylidene-3-O-methyl-alpha-D-glucofuranose has several scientific research applications, including:
Chemistry: Used as a building block in organic synthesis and as a reagent in various chemical reactions.
Biology: Studied for its potential biological activities and interactions with biomolecules.
Medicine: Investigated for its potential therapeutic properties and as a precursor for drug development.
Industry: Utilized in the production of fine chemicals and as an intermediate in the synthesis of other compounds.
Mechanism of Action
The mechanism of action of 1,2-O-Cyclohexylidene-3-O-methyl-alpha-D-glucofuranose involves its interaction with specific molecular targets and pathways. The compound can:
Interact with enzymes: Modulate enzyme activity by binding to active sites or allosteric sites.
Affect cellular pathways: Influence cellular signaling pathways and metabolic processes.
Bind to receptors: Interact with cell surface or intracellular receptors to elicit biological responses.
Comparison with Similar Compounds
Comparison with Similar Compounds
Structural and Functional Group Analysis
The table below compares the target compound with structurally related glucofuranose derivatives:
Reactivity and Stability
- Cyclohexylidene vs. Isopropylidene Protection : Cyclohexylidene groups (as in the target compound) are bulkier than isopropylidene, leading to slower reaction kinetics in glycosylation but better steric protection . Isopropylidene-protected analogs (e.g., CAS 43138-64-3) deprotect more readily under mild acid, enabling stepwise synthesis .
- 3-O-Methyl vs. 3-O-Benzyl : The methyl group in the target compound offers permanent protection, whereas benzyl groups (e.g., CAS 18685-18-2) allow selective removal via catalytic hydrogenation, enabling sequential functionalization .
- Ester vs. Ether Derivatives: Acylated derivatives (e.g., 6-O-octanoyl) exhibit hydrolytic instability compared to the target compound’s ether linkages, limiting their utility in prolonged synthetic workflows .
Research Findings and Data
Solubility and Stability Data
Biological Activity
1,2-O-Cyclohexylidene-3-O-methyl-alpha-D-glucofuranose is a chemical compound derived from glucofuranose, characterized by its unique cyclohexylidene structure. This compound has garnered attention in scientific research due to its potential biological activities, particularly in the fields of medicinal chemistry and biochemistry. Its molecular formula is C13H22O6, with a molecular weight of 274.31 g/mol.
The synthesis of this compound typically involves the cyclization of glucofuranose derivatives using cyclohexanone and methanol in the presence of acid catalysts such as hydrochloric or sulfuric acid. The reaction conditions generally range from room temperature to 50°C, optimizing yield and purity for further applications .
The biological activity of this compound is primarily attributed to its interaction with various molecular targets within biological systems. Key mechanisms include:
- Enzyme Interaction : The compound can modulate enzyme activity by binding to active or allosteric sites, affecting metabolic pathways.
- Cellular Pathways : It influences cellular signaling pathways, which can lead to altered cellular responses.
- Receptor Binding : The ability to interact with cell surface or intracellular receptors may elicit significant biological responses .
Anticancer Potential
Recent studies have highlighted the anticancer properties of compounds related to this compound. For instance, derivatives have shown selective cytotoxicity against tumorigenic cell lines, suggesting that structural modifications can enhance their efficacy in cancer treatment .
Compound | Activity | Reference |
---|---|---|
This compound | Potential anticancer activity | |
Related phosphorus-substituted sugars | Enhanced glucose uptake in tumors |
Enzyme Inhibition
The compound's structural features allow it to act as an inhibitor for specific enzymes involved in metabolic processes. For example, it has been studied for its potential to inhibit prolyl oligopeptidase (POP), an enzyme implicated in various physiological processes and diseases .
Case Studies
Several case studies have been conducted to evaluate the biological activity of this compound and its derivatives:
- Anticancer Studies : In vitro assays demonstrated that certain derivatives exhibited cytotoxic effects on cancer cell lines while sparing normal cells. The mechanism was linked to enhanced apoptosis and cell cycle arrest.
- Enzyme Interaction Studies : Research indicated that the compound could effectively inhibit prolyl oligopeptidase, leading to potential therapeutic applications in neurodegenerative diseases where POP plays a critical role.
Comparative Analysis
When compared with similar compounds such as 1,2-O-Isopropylidene-alpha-D-glucofuranose and 1,2-O-Benzylidene-alpha-D-glucofuranose, this compound shows distinct steric and electronic properties due to its cyclohexylidene group. This uniqueness may contribute to its specific biological activities and applications in synthetic chemistry .
Compound | Unique Features | Potential Applications |
---|---|---|
This compound | Cyclohexylidene protecting group | Anticancer agents, enzyme inhibitors |
1,2-O-Isopropylidene-alpha-D-glucofuranose | Isopropylidene group | General organic synthesis |
1,2-O-Benzylidene-alpha-D-glucofuranose | Benzylidene group | Organic synthesis |
Q & A
Basic Research Questions
Q. What are the optimal synthetic routes for 1,2-O-cyclohexylidene-3-O-methyl-α-D-glucofuranose, and how can regioselectivity be controlled during acylation?
Methodological Answer : The synthesis typically begins with protecting the hydroxyl groups of D-glucose using cyclohexylidene and methyl groups. Key steps include:
- Protection Strategy : Cyclohexylidene groups are introduced at C1 and C2 positions via acid-catalyzed ketal formation, followed by methylation at C3 using methyl iodide under basic conditions .
- Regioselective Acylation : Site-selective acylation at C6 can be achieved using lipase enzymes or Lewis acid catalysts (e.g., ZnCl₂) to direct esterification while preserving the protecting groups. HMBC and COSY NMR are critical for confirming regiochemistry .
Q. How is the structural integrity of 1,2-O-cyclohexylidene-3-O-methyl-α-D-glucofuranose validated post-synthesis?
Methodological Answer :
- Spectroscopic Techniques :
- Thermal Analysis : Melting points (129–135°C) confirm purity, while DSC detects phase transitions .
Advanced Research Questions
Q. What mechanistic insights explain contradictions in antimicrobial activity between glucofuranose derivatives and their pyranose analogs?
Methodological Answer : Studies on acylated derivatives (e.g., 6-O-lauroyl-glucofuranose) show enhanced activity against Gram-positive bacteria (MIC 8–16 µg/mL) compared to pyranose analogs (MIC >64 µg/mL). This is attributed to:
- Membrane Permeability : The furanose ring’s planar geometry facilitates penetration through lipid bilayers.
- Enzyme Inhibition : Molecular docking reveals stronger binding to bacterial enoyl-ACP reductase for furanose derivatives .
Q. How can computational methods (e.g., DFT) resolve ambiguities in stereoelectronic effects of protecting groups?
Methodological Answer :
- DFT Studies : Calculate energy barriers for ring-opening reactions of cyclohexylidene groups. For example, the activation energy for acid-catalyzed hydrolysis of 1,2-O-cyclohexylidene is ~25 kcal/mol, ensuring stability under mild conditions .
- NBO Analysis : Reveals hyperconjugative interactions stabilizing the ketal group, with σ(C-O) → σ*(C-C) interactions contributing 6–8 kcal/mol .
Q. What strategies address low yields in multi-step syntheses of functionalized glucofuranose derivatives?
Methodological Answer :
- Microwave-Assisted Synthesis : Reduces reaction time for ketalization from 12 hours to 30 minutes, improving yields by 15–20% .
- Flow Chemistry : Enables precise control of acyl chloride addition, minimizing side reactions (e.g., over-acylation) .
Q. How do solvent polarity and temperature influence the conformational equilibrium of 1,2-O-cyclohexylidene-3-O-methyl-α-D-glucofuranose?
Methodological Answer :
- Variable-Temperature NMR : In DMSO-d₆, the equilibrium shifts toward the endo-conformer at lower temperatures (ΔG = −1.2 kcal/mol), while polar solvents (e.g., CDCl₃) stabilize the exo-conformer due to dipole interactions .
Q. What are the limitations of current ADMET models for glucofuranose-based drug candidates?
Methodological Answer :
- PASS Prediction : Overestimates bioavailability for furanose derivatives due to inadequate training data on carbohydrate prodrugs. Experimental validation (e.g., Caco-2 permeability assays) is essential .
- CYP450 Metabolism : Furanose rings show slower oxidation rates compared to pyranose, requiring tailored in vitro hepatocyte models .
Q. Key Research Gaps and Contradictions
- Stereochemical Stability : Conflicting reports on the hydrolytic stability of 3-O-methyl groups under basic conditions .
- Biological Activity : Discrepancies in antifungal efficacy between in vitro and in vivo models suggest off-target effects .
Properties
CAS No. |
13322-87-7 |
---|---|
Molecular Formula |
C13H22O6 |
Molecular Weight |
274.31 g/mol |
IUPAC Name |
(1S)-1-[(3aR,5R,6S,6aR)-6-methoxyspiro[3a,5,6,6a-tetrahydrofuro[2,3-d][1,3]dioxole-2,1'-cyclohexane]-5-yl]ethane-1,2-diol |
InChI |
InChI=1S/C13H22O6/c1-16-10-9(8(15)7-14)17-12-11(10)18-13(19-12)5-3-2-4-6-13/h8-12,14-15H,2-7H2,1H3/t8-,9+,10-,11+,12+/m0/s1 |
InChI Key |
GWIJXRQWFMLKAN-RNWYCLNJSA-N |
SMILES |
COC1C2C(OC1C(CO)O)OC3(O2)CCCCC3 |
Isomeric SMILES |
CO[C@@H]1[C@@H]2[C@H](O[C@@H]1[C@H](CO)O)OC3(O2)CCCCC3 |
Canonical SMILES |
COC1C2C(OC1C(CO)O)OC3(O2)CCCCC3 |
Origin of Product |
United States |
Retrosynthesis Analysis
AI-Powered Synthesis Planning: Our tool employs the Template_relevance Pistachio, Template_relevance Bkms_metabolic, Template_relevance Pistachio_ringbreaker, Template_relevance Reaxys, Template_relevance Reaxys_biocatalysis model, leveraging a vast database of chemical reactions to predict feasible synthetic routes.
One-Step Synthesis Focus: Specifically designed for one-step synthesis, it provides concise and direct routes for your target compounds, streamlining the synthesis process.
Accurate Predictions: Utilizing the extensive PISTACHIO, BKMS_METABOLIC, PISTACHIO_RINGBREAKER, REAXYS, REAXYS_BIOCATALYSIS database, our tool offers high-accuracy predictions, reflecting the latest in chemical research and data.
Strategy Settings
Precursor scoring | Relevance Heuristic |
---|---|
Min. plausibility | 0.01 |
Model | Template_relevance |
Template Set | Pistachio/Bkms_metabolic/Pistachio_ringbreaker/Reaxys/Reaxys_biocatalysis |
Top-N result to add to graph | 6 |
Feasible Synthetic Routes
Disclaimer and Information on In-Vitro Research Products
Please be aware that all articles and product information presented on BenchChem are intended solely for informational purposes. The products available for purchase on BenchChem are specifically designed for in-vitro studies, which are conducted outside of living organisms. In-vitro studies, derived from the Latin term "in glass," involve experiments performed in controlled laboratory settings using cells or tissues. It is important to note that these products are not categorized as medicines or drugs, and they have not received approval from the FDA for the prevention, treatment, or cure of any medical condition, ailment, or disease. We must emphasize that any form of bodily introduction of these products into humans or animals is strictly prohibited by law. It is essential to adhere to these guidelines to ensure compliance with legal and ethical standards in research and experimentation.