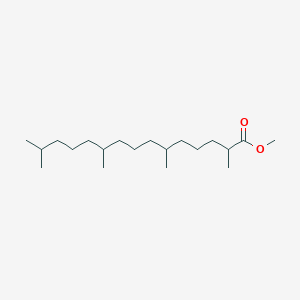
Methyl pristanate
Overview
Description
Methyl pristanate, also known as methyl 2,6,10,14-tetramethylpentadecanoate, is a methyl-branched fatty acid ester. It is derived from pristanic acid, which is a naturally occurring fatty acid found in various marine organisms and certain plant sources. This compound is a colorless liquid with a molecular formula of C20H40O2 and a molecular weight of 312.53 g/mol .
Preparation Methods
Synthetic Routes and Reaction Conditions: Methyl pristanate is typically synthesized through the esterification of pristanic acid with methanol. The reaction is catalyzed by an acid catalyst such as sulfuric acid or methanesulfonic acid. The general reaction conditions involve heating the mixture under reflux to facilitate the esterification process. The reaction can be represented as follows:
Pristanic acid+MethanolAcid catalystMethyl pristanate+Water
Industrial Production Methods: In industrial settings, the production of this compound follows similar principles but on a larger scale. The process involves continuous esterification in a reactor, followed by separation and purification steps such as distillation or extraction to obtain high-purity this compound .
Chemical Reactions Analysis
Types of Reactions: Methyl pristanate undergoes various chemical reactions, including:
Oxidation: this compound can be oxidized to form pristanic acid or other oxidized derivatives.
Reduction: It can be reduced to form the corresponding alcohol.
Substitution: this compound can undergo substitution reactions, particularly at the ester group.
Common Reagents and Conditions:
Oxidation: Common oxidizing agents include potassium permanganate and chromium trioxide.
Reduction: Reducing agents such as lithium aluminum hydride or sodium borohydride are used.
Substitution: Acidic or basic conditions can facilitate substitution reactions.
Major Products:
Oxidation: Pristanic acid.
Reduction: Methyl 2,6,10,14-tetramethylpentadecanol.
Substitution: Various substituted esters depending on the reagents used
Scientific Research Applications
Methyl pristanate has several applications in scientific research:
Chemistry: It is used as an intermediate in the synthesis of other complex organic molecules.
Biology: this compound is studied for its role in metabolic pathways, particularly in the context of peroxisomal disorders.
Medicine: Research is ongoing to explore its potential therapeutic applications, especially in the treatment of metabolic diseases.
Industry: It is used as a lubricant additive due to its chemical stability and thermal properties
Mechanism of Action
Methyl pristanate exerts its effects primarily through its metabolism in the body. It is metabolized to pristanic acid, which then undergoes further metabolic processes. The key molecular targets include enzymes involved in fatty acid oxidation, such as acyl-CoA oxidase and peroxisomal acyl-CoA thioesterase. These enzymes play crucial roles in the breakdown and utilization of fatty acids .
Comparison with Similar Compounds
Pristanic Acid: The parent compound of methyl pristanate, involved in similar metabolic pathways.
Phytanic Acid: Another methyl-branched fatty acid with similar metabolic roles but different structural features.
Methyl Heptadecanoate: A straight-chain fatty acid ester with different physical and chemical properties.
Uniqueness: this compound is unique due to its branched structure, which imparts distinct chemical and physical properties compared to straight-chain fatty acid esters. This branching affects its reactivity, solubility, and thermal stability, making it valuable in specific industrial and research applications .
Properties
IUPAC Name |
methyl 2,6,10,14-tetramethylpentadecanoate | |
---|---|---|
Source | PubChem | |
URL | https://pubchem.ncbi.nlm.nih.gov | |
Description | Data deposited in or computed by PubChem | |
InChI |
InChI=1S/C20H40O2/c1-16(2)10-7-11-17(3)12-8-13-18(4)14-9-15-19(5)20(21)22-6/h16-19H,7-15H2,1-6H3 | |
Source | PubChem | |
URL | https://pubchem.ncbi.nlm.nih.gov | |
Description | Data deposited in or computed by PubChem | |
InChI Key |
LCWYDFQCGCPILG-UHFFFAOYSA-N | |
Source | PubChem | |
URL | https://pubchem.ncbi.nlm.nih.gov | |
Description | Data deposited in or computed by PubChem | |
Canonical SMILES |
CC(C)CCCC(C)CCCC(C)CCCC(C)C(=O)OC | |
Source | PubChem | |
URL | https://pubchem.ncbi.nlm.nih.gov | |
Description | Data deposited in or computed by PubChem | |
Molecular Formula |
C20H40O2 | |
Source | PubChem | |
URL | https://pubchem.ncbi.nlm.nih.gov | |
Description | Data deposited in or computed by PubChem | |
DSSTOX Substance ID |
DTXSID80336819 | |
Record name | Methyl pristanate | |
Source | EPA DSSTox | |
URL | https://comptox.epa.gov/dashboard/DTXSID80336819 | |
Description | DSSTox provides a high quality public chemistry resource for supporting improved predictive toxicology. | |
Molecular Weight |
312.5 g/mol | |
Source | PubChem | |
URL | https://pubchem.ncbi.nlm.nih.gov | |
Description | Data deposited in or computed by PubChem | |
CAS No. |
1001-80-5 | |
Record name | Methyl pristanate | |
Source | EPA DSSTox | |
URL | https://comptox.epa.gov/dashboard/DTXSID80336819 | |
Description | DSSTox provides a high quality public chemistry resource for supporting improved predictive toxicology. | |
Retrosynthesis Analysis
AI-Powered Synthesis Planning: Our tool employs the Template_relevance Pistachio, Template_relevance Bkms_metabolic, Template_relevance Pistachio_ringbreaker, Template_relevance Reaxys, Template_relevance Reaxys_biocatalysis model, leveraging a vast database of chemical reactions to predict feasible synthetic routes.
One-Step Synthesis Focus: Specifically designed for one-step synthesis, it provides concise and direct routes for your target compounds, streamlining the synthesis process.
Accurate Predictions: Utilizing the extensive PISTACHIO, BKMS_METABOLIC, PISTACHIO_RINGBREAKER, REAXYS, REAXYS_BIOCATALYSIS database, our tool offers high-accuracy predictions, reflecting the latest in chemical research and data.
Strategy Settings
Precursor scoring | Relevance Heuristic |
---|---|
Min. plausibility | 0.01 |
Model | Template_relevance |
Template Set | Pistachio/Bkms_metabolic/Pistachio_ringbreaker/Reaxys/Reaxys_biocatalysis |
Top-N result to add to graph | 6 |
Feasible Synthetic Routes
Q1: What is the role of methyl pristanate in the synthesis of head-to-head isoprenoid hydrocarbons?
A1: this compound [] serves as a starting material in the synthesis of head-to-head isoprenoid hydrocarbons, specifically iC19-iC19. The synthesis involves a three-step route starting with this compound, converting it to pristanol, then to the corresponding bromide, and finally to the target iC19-iC19 hydrocarbon [].
Disclaimer and Information on In-Vitro Research Products
Please be aware that all articles and product information presented on BenchChem are intended solely for informational purposes. The products available for purchase on BenchChem are specifically designed for in-vitro studies, which are conducted outside of living organisms. In-vitro studies, derived from the Latin term "in glass," involve experiments performed in controlled laboratory settings using cells or tissues. It is important to note that these products are not categorized as medicines or drugs, and they have not received approval from the FDA for the prevention, treatment, or cure of any medical condition, ailment, or disease. We must emphasize that any form of bodily introduction of these products into humans or animals is strictly prohibited by law. It is essential to adhere to these guidelines to ensure compliance with legal and ethical standards in research and experimentation.