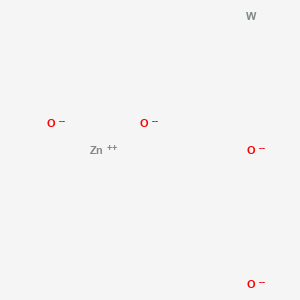
Tungsten zinc tetraoxide
Overview
Description
Tungsten zinc tetraoxide, commonly known as zinc tungstate (ZnWO₄), is an inorganic compound with the chemical formula ZnWO₄ and a molecular weight of 313.218 g/mol . It appears as a white, crystalline powder that is stable under ambient conditions and insoluble in water . Its crystal structure belongs to the monoclinic wolframite type, characterized by alternating layers of ZnO₆ and WO₆ octahedra .
Preparation Methods
Synthetic Routes and Reaction Conditions: Tungsten zinc tetraoxide can be synthesized through various methods, including solid-state reactions, sol-gel processes, and hydrothermal synthesis.
Solid-State Reactions: This method involves mixing tungsten oxide and zinc oxide powders in stoichiometric amounts, followed by heating the mixture at high temperatures (typically above 800°C) to form the desired compound.
Sol-Gel Processes: In this method, tungsten and zinc precursors are dissolved in a solvent to form a homogeneous solution. The solution is then subjected to hydrolysis and condensation reactions to form a gel, which is subsequently dried and calcined to obtain this compound.
Hydrothermal Synthesis: This method involves reacting tungsten and zinc precursors in an aqueous solution at elevated temperatures and pressures. The reaction conditions can be optimized to control the size and morphology of the resulting particles.
Industrial Production Methods: Industrial production of this compound typically involves large-scale solid-state reactions or hydrothermal synthesis. These methods are preferred due to their scalability and ability to produce high-purity materials.
Chemical Reactions Analysis
Types of Reactions: Tungsten zinc tetraoxide can undergo various chemical reactions, including oxidation, reduction, and substitution reactions.
Oxidation: The compound can be oxidized to form higher oxidation state compounds, such as tungsten(VI) oxide and zinc oxide.
Reduction: this compound can be reduced using reducing agents like hydrogen or carbon monoxide to form lower oxidation state compounds.
Substitution: The compound can undergo substitution reactions where one of the metal ions is replaced by another metal ion, leading to the formation of mixed-metal oxides.
Common Reagents and Conditions:
Oxidation: Oxygen or air at elevated temperatures.
Reduction: Hydrogen gas or carbon monoxide at high temperatures.
Substitution: Metal salts in aqueous or non-aqueous solutions under controlled conditions.
Major Products Formed:
Oxidation: Tungsten(VI) oxide and zinc oxide.
Reduction: Lower oxidation state tungsten and zinc compounds.
Substitution: Mixed-metal oxides with varying compositions.
Scientific Research Applications
Physical and Chemical Properties
- Molecular Formula : WZnO
- Molar Mass : Approximately 303.8 g/mol
- Density : Varies depending on the synthesis method
- Band Gap : Typically around 2.6 eV, making it suitable for optoelectronic applications.
Semiconductor Devices
Tungsten zinc tetraoxide is used as a semiconductor material in various electronic devices. Its properties allow for effective charge transport, making it suitable for use in:
- Thin Film Transistors (TFTs) : The compound can be employed as an active layer in TFTs, enhancing device performance due to its high mobility.
- Photodetectors : Its sensitivity to light makes it valuable in photodetection applications.
Solar Cells
Recent studies have explored the use of this compound as an electron transport layer in perovskite solar cells. The compound improves the efficiency and stability of these cells by optimizing surface morphology and electronic properties .
Environmental Catalysis
This compound has shown promise as a catalyst for various environmental applications:
- DeNOx Catalysis : It is effective in the selective catalytic reduction (SCR) of nitrogen oxides (NOx), which are harmful pollutants generated from combustion processes.
- Photocatalysis : The compound can be used in photocatalytic reactions to degrade organic pollutants under UV or visible light, leveraging its semiconductor properties .
Industrial Catalysis
In industrial settings, this compound serves as a catalyst for:
- Hydrocarbon Processing : Its catalytic activity can facilitate reactions such as hydrocracking and reforming, improving yield and efficiency in petrochemical processes.
Coatings
The compound is utilized in protective coatings due to its corrosion resistance and durability. This compound coatings are applied in:
- Aerospace Components : Enhancing the lifespan and performance of critical components exposed to harsh environments.
- Automotive Parts : Providing wear resistance and protecting against corrosion.
Composite Materials
In composite materials, this compound can be integrated to improve mechanical properties such as strength and toughness, making it suitable for applications in:
- Construction Materials : Enhancing the performance of concrete and other building materials.
- Sports Equipment : Improving the durability of equipment subjected to high stress.
Case Study: Photocatalytic Degradation of Pollutants
A study demonstrated that this compound effectively degraded methylene blue dye under UV light exposure, showcasing its potential for wastewater treatment applications . The degradation efficiency was found to be over 90% within 60 minutes, indicating its effectiveness as a photocatalyst.
Case Study: Performance in Solar Cells
Research indicated that incorporating this compound as an electron transport layer in perovskite solar cells resulted in an increase in power conversion efficiency from 18% to 22%, highlighting its role in enhancing solar energy conversion technologies .
Mechanism of Action
The mechanism of action of tungsten zinc tetraoxide depends on its specific application.
Catalysis: In catalytic applications, the compound acts as a catalyst by providing active sites for chemical reactions to occur. The presence of both tungsten and zinc oxides can enhance the catalytic activity and selectivity of the compound.
Photothermal Therapy: In photothermal therapy, this compound absorbs light and converts it into heat, which can be used to destroy cancer cells. The compound’s ability to absorb light in the near-infrared region makes it particularly effective for this application.
Electronic Applications: In electronic applications, this compound can improve the performance of electronic devices by enhancing their thermal stability and electronic properties.
Comparison with Similar Compounds
Key Properties:
- Band Gap : ZnWO₄ exhibits a direct band gap of 4.6–5.4 eV, as confirmed by experimental and computational studies .
- Melting Point : 1220°C .
- Applications : Used in optical devices, photocatalysis, heavy metal wastewater treatment (as an adsorbent), and radiation detection .
Transition Metal Tungstates (NiWO₄, FeWO₄, CoWO₄, CuWO₄)
These tungstates share the general formula MWO₄ (M = transition metal) and wolframite-like structures but differ in electronic and optical properties due to their metal cation configurations:
Key Insight : ZnWO₄ and CdWO₄ exhibit direct band gaps due to their d¹⁰ cations, making them suitable for optoelectronic applications. In contrast, NiWO₄ and FeWO₄ have indirect gaps influenced by partially filled d-orbitals, favoring catalytic and magnetic uses .
Zinc Molybdate (ZnMoO₄)
ZnMoO₄ shares a similar structure with ZnWO₄ but replaces tungsten with molybdenum:
Key Insight : The smaller ionic radius of Mo⁶⁶⁺ compared to W⁶⁶⁺ reduces ZnMoO₄’s thermal stability but enhances its photocatalytic activity under visible light .
Diiron Zinc Tetraoxide (Fe₂ZnO₄)
Fe₂ZnO₄ is a mixed-metal oxide with distinct structural and functional properties:
Property | ZnWO₄ | Fe₂ZnO₄ |
---|---|---|
Composition | Zn²⁺ + WO₄²⁻ | Fe³⁺ + Zn²⁺ + O²⁻ |
Structure | Monoclinic | Spinel cubic |
Applications | Adsorption, optics | Magnetic storage, pigments |
Band Gap | 4.6–5.4 eV | ~2.1 eV |
References |
Key Insight: Fe₂ZnO₄’s spinel structure and lower band gap enable applications in magnetism and electronics, contrasting with ZnWO₄’s optical uses .
Q & A
Basic Research Questions
Q. How can researchers verify the purity and crystallinity of synthesized tungsten zinc tetraoxide (ZnWO₄)?
To confirm structural integrity, use X-ray diffraction (XRD) to match peaks with reference patterns (JCPDS card 15-0774 for ZnWO₄). Pair with scanning/transmission electron microscopy (SEM/TEM) to analyze morphology and energy-dispersive X-ray spectroscopy (EDX) for elemental composition validation. Thermogravimetric analysis (TGA) can assess thermal stability and phase transitions .
Q. What are the primary challenges in synthesizing phase-pure ZnWO₄ nanoparticles?
Common issues include incomplete solid-state reactions between ZnO and WO₃ precursors, leading to impurities like unreacted oxides. Optimize synthesis parameters (e.g., hydrothermal conditions: 180–200°C for 12–24 hours) to enhance crystallinity. Use Rietveld refinement of XRD data to quantify phase purity .
Q. What are the key considerations for safe handling and storage of ZnWO₄ in laboratory environments?
ZnWO₄ is stable under ambient conditions but may release toxic tungsten oxides if heated above 1000°C. Store in airtight containers away from strong acids/oxidizers. Use standard personal protective equipment (PPE) and fume hoods during high-temperature processing .
Advanced Research Questions
Q. How does the electronic band structure of ZnWO₄ influence its photocatalytic performance?
ZnWO₄ exhibits a direct bandgap (~4.6–5.4 eV), with valence bands dominated by hybridized O 2p and Zn 3d orbitals. The conduction band comprises W 5d states, facilitating electron-hole pair separation under UV irradiation. Tailor bandgap via doping (e.g., Fe³⁺ or Mo⁶⁺) to enhance visible-light absorption .
Q. How can computational methods like DFT be applied to predict the electronic properties of ZnWO₄?
Density functional theory (DFT) with hybrid functionals (e.g., HSE06) accurately models ZnWO₄’s band structure. Compare computed bandgap values (4.6 eV) with experimental data from UV-Vis diffuse reflectance spectroscopy and ultraviolet photoelectron spectroscopy (UPS) . Discrepancies often arise from excitonic effects or surface defects .
Q. What advanced applications of ZnWO₄ are emerging in materials science?
- Photocatalysis : Degrade organic pollutants (e.g., methylene blue) under UV light via reactive oxygen species generation.
- Scintillators : High-density ZnWO₄ crystals detect gamma rays in nuclear physics experiments.
- Electrodes : Composite ZnWO₄/CNT structures show promise in supercapacitors due to redox-active W centers .
Q. How should researchers address discrepancies between theoretical and experimental bandgap values in ZnWO₄?
Reconcile differences by:
- Validating computational parameters (e.g., k-point mesh, exchange-correlation functionals).
- Accounting for experimental conditions (e.g., surface roughness, oxygen vacancies).
- Cross-referencing with ellipsometry or photoluminescence spectroscopy for precise optical measurements .
Q. What methodologies are effective in analyzing ZnWO₄’s thermal decomposition pathways?
Use thermogravimetric analysis-mass spectrometry (TGA-MS) to identify gaseous byproducts (e.g., WO₃ sublimation above 1100°C). Pair with in situ XRD to track phase transitions and kinetic studies via the Kissinger method to determine activation energies .
Q. How does ZnWO₄’s crystal morphology impact its functional properties?
Nanostructured ZnWO₄ (e.g., nanorods) offers higher surface area for catalytic reactions, while single crystals minimize defect-related charge recombination. Control morphology via surfactant-assisted hydrothermal synthesis (e.g., cetyltrimethylammonium bromide) .
Q. What spectroscopic techniques are critical for probing ZnWO₄’s defect chemistry?
- Raman spectroscopy : Identify vibrational modes (e.g., W–O stretching at ~880 cm⁻¹) to detect oxygen vacancies.
- X-ray photoelectron spectroscopy (XPS) : Quantify oxidation states (W⁶⁺, Zn²⁺) and surface adsorbates .
Q. Key Research Recommendations
- Explore ZnWO₄’s potential in photoelectrochemical water splitting by coupling with narrow-bandgap semiconductors (e.g., TiO₂).
- Investigate dopant-induced magnetism for spintronic applications using rare-earth elements (e.g., Eu³⁺).
- Optimize solvothermal synthesis to achieve sub-50 nm particles for enhanced catalytic activity.
Properties
IUPAC Name |
zinc;oxygen(2-);tungsten | |
---|---|---|
Source | PubChem | |
URL | https://pubchem.ncbi.nlm.nih.gov | |
Description | Data deposited in or computed by PubChem | |
InChI |
InChI=1S/4O.W.Zn/q4*-2;;+2 | |
Source | PubChem | |
URL | https://pubchem.ncbi.nlm.nih.gov | |
Description | Data deposited in or computed by PubChem | |
InChI Key |
IGKCQWHBCHMDFW-UHFFFAOYSA-N | |
Source | PubChem | |
URL | https://pubchem.ncbi.nlm.nih.gov | |
Description | Data deposited in or computed by PubChem | |
Canonical SMILES |
[O-2].[O-2].[O-2].[O-2].[Zn+2].[W] | |
Source | PubChem | |
URL | https://pubchem.ncbi.nlm.nih.gov | |
Description | Data deposited in or computed by PubChem | |
Molecular Formula |
O4WZn-6 | |
Source | PubChem | |
URL | https://pubchem.ncbi.nlm.nih.gov | |
Description | Data deposited in or computed by PubChem | |
DSSTOX Substance ID |
DTXSID401014402 | |
Record name | Tungsten zinc oxide (WZnO4) | |
Source | EPA DSSTox | |
URL | https://comptox.epa.gov/dashboard/DTXSID401014402 | |
Description | DSSTox provides a high quality public chemistry resource for supporting improved predictive toxicology. | |
Molecular Weight |
313.2 g/mol | |
Source | PubChem | |
URL | https://pubchem.ncbi.nlm.nih.gov | |
Description | Data deposited in or computed by PubChem | |
CAS No. |
13597-56-3 | |
Record name | Tungsten zinc oxide (WZnO4) | |
Source | ChemIDplus | |
URL | https://pubchem.ncbi.nlm.nih.gov/substance/?source=chemidplus&sourceid=0013597563 | |
Description | ChemIDplus is a free, web search system that provides access to the structure and nomenclature authority files used for the identification of chemical substances cited in National Library of Medicine (NLM) databases, including the TOXNET system. | |
Record name | Tungsten zinc oxide (WZnO4) | |
Source | EPA Chemicals under the TSCA | |
URL | https://www.epa.gov/chemicals-under-tsca | |
Description | EPA Chemicals under the Toxic Substances Control Act (TSCA) collection contains information on chemicals and their regulations under TSCA, including non-confidential content from the TSCA Chemical Substance Inventory and Chemical Data Reporting. | |
Record name | Tungsten zinc oxide (WZnO4) | |
Source | EPA DSSTox | |
URL | https://comptox.epa.gov/dashboard/DTXSID401014402 | |
Description | DSSTox provides a high quality public chemistry resource for supporting improved predictive toxicology. | |
Record name | Tungsten zinc tetraoxide | |
Source | European Chemicals Agency (ECHA) | |
URL | https://echa.europa.eu/substance-information/-/substanceinfo/100.033.670 | |
Description | The European Chemicals Agency (ECHA) is an agency of the European Union which is the driving force among regulatory authorities in implementing the EU's groundbreaking chemicals legislation for the benefit of human health and the environment as well as for innovation and competitiveness. | |
Explanation | Use of the information, documents and data from the ECHA website is subject to the terms and conditions of this Legal Notice, and subject to other binding limitations provided for under applicable law, the information, documents and data made available on the ECHA website may be reproduced, distributed and/or used, totally or in part, for non-commercial purposes provided that ECHA is acknowledged as the source: "Source: European Chemicals Agency, http://echa.europa.eu/". Such acknowledgement must be included in each copy of the material. ECHA permits and encourages organisations and individuals to create links to the ECHA website under the following cumulative conditions: Links can only be made to webpages that provide a link to the Legal Notice page. | |
Disclaimer and Information on In-Vitro Research Products
Please be aware that all articles and product information presented on BenchChem are intended solely for informational purposes. The products available for purchase on BenchChem are specifically designed for in-vitro studies, which are conducted outside of living organisms. In-vitro studies, derived from the Latin term "in glass," involve experiments performed in controlled laboratory settings using cells or tissues. It is important to note that these products are not categorized as medicines or drugs, and they have not received approval from the FDA for the prevention, treatment, or cure of any medical condition, ailment, or disease. We must emphasize that any form of bodily introduction of these products into humans or animals is strictly prohibited by law. It is essential to adhere to these guidelines to ensure compliance with legal and ethical standards in research and experimentation.