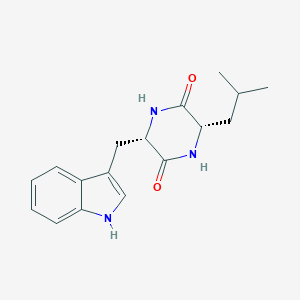
Cyclo(L-leucyl-L-tryptophyl)
Overview
Description
. This compound is a cyclic dipeptide formed by the condensation of the amino acids leucine and tryptophan. Diketopiperazines are known for their diverse biological activities, including antibacterial, antifungal, and antiviral properties .
Preparation Methods
Synthetic Routes and Reaction Conditions: Cyclo(L-Leu-L-Trp) can be synthesized through various methods, including chemical synthesis and biosynthesis. One common method involves the use of cyclodipeptide synthases (CDPSs), which are enzymes that catalyze the formation of cyclic dipeptides from aminoacyl-tRNAs . In this process, the CDPSs hijack aminoacyl-tRNAs from the ribosomal machinery to form the cyclic dipeptide.
Industrial Production Methods: Industrial production of Cyclo(L-Leu-L-Trp) can be achieved through fermentation processes using microorganisms such as Penicillium. The culture conditions, including the medium composition and fermentation parameters, are optimized to maximize the yield of the desired compound .
Chemical Reactions Analysis
Types of Reactions: Cyclo(L-Leu-L-Trp) undergoes various chemical reactions, including oxidation, reduction, and substitution reactions. These reactions can be used to modify the compound and enhance its biological activity.
Common Reagents and Conditions:
Oxidation: Common oxidizing agents such as hydrogen peroxide or potassium permanganate can be used to oxidize Cyclo(L-Leu-L-Trp).
Reduction: Reducing agents like sodium borohydride or lithium aluminum hydride can be employed to reduce the compound.
Substitution: Substitution reactions can be carried out using reagents like halogens or alkylating agents.
Major Products: The major products formed from these reactions depend on the specific reagents and conditions used. For example, oxidation of Cyclo(L-Leu-L-Trp) can lead to the formation of hydroxylated derivatives, while reduction can yield reduced diketopiperazine analogs .
Scientific Research Applications
Cyclo(L-Leu-L-Trp) has a wide range of scientific research applications, including:
Mechanism of Action
The mechanism of action of Cyclo(L-Leu-L-Trp) involves its interaction with specific molecular targets and pathways. For example, as a melatonin receptor agonist, it binds to melatonin receptors and inhibits the accumulation of cyclic adenosine monophosphate (cAMP), leading to various physiological effects . Additionally, its antibacterial and antifungal activities are attributed to its ability to disrupt microbial cell membranes and inhibit essential enzymes .
Comparison with Similar Compounds
Cyclo(L-Leu-L-Trp) can be compared with other similar cyclic dipeptides, such as:
Cyclo(L-Trp-L-Trp): This compound is also a diketopiperazine with antibacterial activity, but it differs in its amino acid composition and specific biological activities.
Cyclo(L-Trp-L-Pro): Another diketopiperazine with distinct structural and biological properties.
Cyclo(L-Leu-L-Leu): A cyclic dipeptide composed of two leucine residues, exhibiting different biological activities compared to Cyclo(L-Leu-L-Trp).
The uniqueness of Cyclo(L-Leu-L-Trp) lies in its specific combination of leucine and tryptophan, which imparts distinct biological activities and potential therapeutic applications .
Properties
IUPAC Name |
(3S,6S)-3-(1H-indol-3-ylmethyl)-6-(2-methylpropyl)piperazine-2,5-dione | |
---|---|---|
Source | PubChem | |
URL | https://pubchem.ncbi.nlm.nih.gov | |
Description | Data deposited in or computed by PubChem | |
InChI |
InChI=1S/C17H21N3O2/c1-10(2)7-14-16(21)20-15(17(22)19-14)8-11-9-18-13-6-4-3-5-12(11)13/h3-6,9-10,14-15,18H,7-8H2,1-2H3,(H,19,22)(H,20,21)/t14-,15-/m0/s1 | |
Source | PubChem | |
URL | https://pubchem.ncbi.nlm.nih.gov | |
Description | Data deposited in or computed by PubChem | |
InChI Key |
BZUNCDPEEKFTCX-GJZGRUSLSA-N | |
Source | PubChem | |
URL | https://pubchem.ncbi.nlm.nih.gov | |
Description | Data deposited in or computed by PubChem | |
Canonical SMILES |
CC(C)CC1C(=O)NC(C(=O)N1)CC2=CNC3=CC=CC=C32 | |
Source | PubChem | |
URL | https://pubchem.ncbi.nlm.nih.gov | |
Description | Data deposited in or computed by PubChem | |
Isomeric SMILES |
CC(C)C[C@H]1C(=O)N[C@H](C(=O)N1)CC2=CNC3=CC=CC=C32 | |
Source | PubChem | |
URL | https://pubchem.ncbi.nlm.nih.gov | |
Description | Data deposited in or computed by PubChem | |
Molecular Formula |
C17H21N3O2 | |
Source | PubChem | |
URL | https://pubchem.ncbi.nlm.nih.gov | |
Description | Data deposited in or computed by PubChem | |
DSSTOX Substance ID |
DTXSID10164768 | |
Record name | Cyclo(L-leucyl-L-tryptophyl) | |
Source | EPA DSSTox | |
URL | https://comptox.epa.gov/dashboard/DTXSID10164768 | |
Description | DSSTox provides a high quality public chemistry resource for supporting improved predictive toxicology. | |
Molecular Weight |
299.37 g/mol | |
Source | PubChem | |
URL | https://pubchem.ncbi.nlm.nih.gov | |
Description | Data deposited in or computed by PubChem | |
CAS No. |
15136-34-2 | |
Record name | Cyclo(L-leucyl-L-tryptophyl) | |
Source | ChemIDplus | |
URL | https://pubchem.ncbi.nlm.nih.gov/substance/?source=chemidplus&sourceid=0015136342 | |
Description | ChemIDplus is a free, web search system that provides access to the structure and nomenclature authority files used for the identification of chemical substances cited in National Library of Medicine (NLM) databases, including the TOXNET system. | |
Record name | Cyclo(L-leucyl-L-tryptophyl) | |
Source | EPA DSSTox | |
URL | https://comptox.epa.gov/dashboard/DTXSID10164768 | |
Description | DSSTox provides a high quality public chemistry resource for supporting improved predictive toxicology. | |
Retrosynthesis Analysis
AI-Powered Synthesis Planning: Our tool employs the Template_relevance Pistachio, Template_relevance Bkms_metabolic, Template_relevance Pistachio_ringbreaker, Template_relevance Reaxys, Template_relevance Reaxys_biocatalysis model, leveraging a vast database of chemical reactions to predict feasible synthetic routes.
One-Step Synthesis Focus: Specifically designed for one-step synthesis, it provides concise and direct routes for your target compounds, streamlining the synthesis process.
Accurate Predictions: Utilizing the extensive PISTACHIO, BKMS_METABOLIC, PISTACHIO_RINGBREAKER, REAXYS, REAXYS_BIOCATALYSIS database, our tool offers high-accuracy predictions, reflecting the latest in chemical research and data.
Strategy Settings
Precursor scoring | Relevance Heuristic |
---|---|
Min. plausibility | 0.01 |
Model | Template_relevance |
Template Set | Pistachio/Bkms_metabolic/Pistachio_ringbreaker/Reaxys/Reaxys_biocatalysis |
Top-N result to add to graph | 6 |
Feasible Synthetic Routes
Q1: How does Cyclo(L-leucyl-L-tryptophyl) interact with its target (the bitter taste receptor) and what are the downstream effects?
A1: The research suggests that Cyclo(L-leucyl-L-tryptophyl) interacts with a receptor that doesn't seem to be strictly defined by a specific conformation or chirality of the molecule []. Instead, the research highlights the importance of hydrophobicity in the binding process []. While the exact receptor remains unidentified, experiments point towards a lecithin-like lipid as a potential binding site within the cell membrane []. This binding likely triggers signaling cascades within taste receptor cells, ultimately leading to the perception of bitterness.
Q2: What is known about the structure-activity relationship (SAR) of Cyclo(L-leucyl-L-tryptophyl) and its bitterness?
A2: The study indicates that the bitterness of Cyclo(L-leucyl-L-tryptophyl) and similar diketopiperazines is strongly correlated with their hydrophobicity []. This implies that modifications to the molecule's structure, particularly those impacting its hydrophobicity, could significantly influence its perceived bitterness. For instance, substituting the leucine or tryptophan residues with more hydrophobic amino acids might enhance the bitterness, while introducing hydrophilic groups could potentially diminish it.
Disclaimer and Information on In-Vitro Research Products
Please be aware that all articles and product information presented on BenchChem are intended solely for informational purposes. The products available for purchase on BenchChem are specifically designed for in-vitro studies, which are conducted outside of living organisms. In-vitro studies, derived from the Latin term "in glass," involve experiments performed in controlled laboratory settings using cells or tissues. It is important to note that these products are not categorized as medicines or drugs, and they have not received approval from the FDA for the prevention, treatment, or cure of any medical condition, ailment, or disease. We must emphasize that any form of bodily introduction of these products into humans or animals is strictly prohibited by law. It is essential to adhere to these guidelines to ensure compliance with legal and ethical standards in research and experimentation.