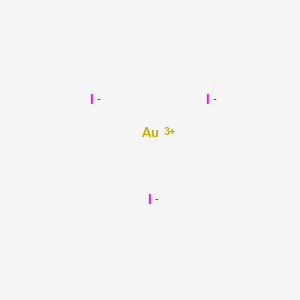
Triiodogold(III)
- Click on QUICK INQUIRY to receive a quote from our team of experts.
- With the quality product at a COMPETITIVE price, you can focus more on your research.
Overview
Mechanism of Action
Target of Action
Gold compounds, including Gold Triiodide, have been shown to target several metabolic pathways and a number of metabolites in tumor cells . In most situations, the gold complexes exhibit anticancer activities by targeting thioredoxin reductase (TrxR) or other thiol-rich proteins and enzymes .
Mode of Action
The halido (NHC)gold(I) complexes, which could be related to Gold Triiodide, react very rapidly with model nucleophiles, e.g., iodide or selenocysteine (Sec). For instance, Sec transformed the complex in a proportion of 73.03% to the (NHC)Au(I)Sec complex during 5 min of incubation . This high reactivity against this amino acid, present in the active site of the thioredoxin reductase (TrxR), correlates with the complete inhibition of the isolated TrxR enzyme at 1 μM .
Biochemical Pathways
Gold compounds have been shown to modulate glucose, protein, and nucleic acid metabolism in tumor cells, resulting in anti-tumor effects . They trigger cell death via reactive oxygen species (ROS) .
Result of Action
The result of Gold Triiodide’s action is the inhibition of the TrxR enzyme, leading to cell death via reactive oxygen species (ROS) . This can have significant anti-tumor effects .
Biochemical Analysis
Biochemical Properties
Gold triiodide plays a crucial role in biochemical reactions due to its ability to interact with various enzymes, proteins, and other biomolecules. The compound’s high affinity for sulfur-containing groups allows it to bind effectively to thiol groups in cysteine residues of proteins. This interaction can lead to the inhibition or activation of enzyme activity, depending on the specific enzyme involved. For instance, gold triiodide has been shown to inhibit thioredoxin reductase, an enzyme involved in maintaining the redox balance within cells . Additionally, gold triiodide can interact with DNA, potentially leading to modifications in gene expression.
Cellular Effects
The effects of gold triiodide on cellular processes are profound. In various cell types, gold triiodide influences cell function by altering cell signaling pathways, gene expression, and cellular metabolism. For example, gold triiodide has been observed to induce apoptosis in cancer cells by disrupting mitochondrial function and activating caspase pathways . This compound also affects the expression of genes involved in oxidative stress responses, leading to increased production of reactive oxygen species (ROS) and subsequent cellular damage. Furthermore, gold triiodide can modulate metabolic pathways by inhibiting key enzymes, thereby affecting cellular energy production and biosynthesis.
Molecular Mechanism
At the molecular level, gold triiodide exerts its effects through several mechanisms. One primary mechanism involves the binding of gold triiodide to thiol groups in proteins, leading to the formation of stable gold-thiol complexes. This binding can result in the inhibition of enzyme activity, as seen with thioredoxin reductase . Additionally, gold triiodide can interact with nucleic acids, causing structural changes that affect gene expression. The compound’s ability to generate ROS also plays a significant role in its molecular mechanism, as increased ROS levels can lead to oxidative damage to cellular components, including lipids, proteins, and DNA .
Temporal Effects in Laboratory Settings
The effects of gold triiodide in laboratory settings can vary over time. The stability of gold triiodide is a critical factor, as it can degrade under certain conditions, leading to changes in its biochemical activity. Studies have shown that gold triiodide remains stable under physiological conditions but can degrade in the presence of strong reducing agents . Long-term exposure to gold triiodide in in vitro and in vivo studies has revealed that the compound can cause sustained oxidative stress, leading to chronic cellular damage and altered cellular functions over time.
Dosage Effects in Animal Models
The effects of gold triiodide in animal models are dose-dependent. At low doses, gold triiodide can exhibit therapeutic effects, such as anti-cancer activity, by inducing apoptosis in tumor cells . At higher doses, the compound can become toxic, leading to adverse effects such as hepatotoxicity and nephrotoxicity. Threshold effects have been observed, where a specific dosage range is required to achieve the desired therapeutic outcome without causing significant toxicity . These findings highlight the importance of careful dosage optimization in the use of gold triiodide for therapeutic applications.
Metabolic Pathways
Gold triiodide is involved in several metabolic pathways, primarily through its interactions with enzymes and cofactors. The compound’s ability to inhibit thioredoxin reductase affects the redox balance within cells, leading to alterations in metabolic processes . Additionally, gold triiodide can interfere with the function of other enzymes involved in cellular metabolism, such as those in the glycolytic pathway and the tricarboxylic acid (TCA) cycle. These interactions can result in changes in cellular energy production and biosynthetic pathways, ultimately affecting cell viability and function.
Preparation Methods
Triiodogold(III) can be synthesized by reacting gold trichloride with potassium iodide solution . The reaction typically involves dissolving gold trichloride in water and then adding an aqueous solution of potassium iodide. The resulting mixture is stirred, leading to the formation of Triiodogold(III) as a precipitate. The precipitate is then filtered, washed, and dried to obtain the final product.
Chemical Reactions Analysis
Triiodogold(III) undergoes various types of chemical reactions, including:
Substitution Reactions: Triiodogold(III) can participate in substitution reactions where the iodide ligands are replaced by other ligands.
Reduction Reactions: Triiodogold(III) can be reduced to gold(I) or elemental gold using reducing agents such as sodium borohydride or hydrazine.
Oxidation Reactions: Although less common, Triiodogold(III) can undergo oxidation reactions under specific conditions, leading to the formation of higher oxidation state gold compounds.
The major products formed from these reactions depend on the specific reagents and conditions used. For example, substitution reactions with phosphines can yield gold-phosphine complexes, while reduction reactions typically produce gold(I) or elemental gold.
Scientific Research Applications
Triiodogold(III) has several scientific research applications, including:
Medicinal Chemistry: Gold(III) complexes, including Triiodogold(III), have shown potential as anticancer agents.
Catalysis: Triiodogold(III) is used as a catalyst in various organic transformations, including electrophilic functional group transfer reactions.
Material Science: Triiodogold(III) is studied for its potential use in the synthesis of gold nanoparticles, which have applications in electronics, photonics, and biomedical fields.
Comparison with Similar Compounds
Triiodogold(III) can be compared with other gold(III) complexes, such as gold(III) chloride and gold(III) bromide. These compounds share similar structural features and reactivity patterns but differ in their specific applications and stability. For example:
Gold(III) Chloride (AuCl₃): This compound is more commonly used in catalysis and material science due to its higher stability and solubility compared to Triiodogold(III).
Gold(III) Bromide (AuBr₃): Similar to Triiodogold(III), gold(III) bromide is used in organic synthesis and medicinal chemistry.
These comparisons highlight the uniqueness of Triiodogold(III) in terms of its specific reactivity and applications.
Properties
CAS No. |
13453-24-2 |
---|---|
Molecular Formula |
AuI3 |
Molecular Weight |
577.6800 g/mol |
IUPAC Name |
triiodogold |
InChI |
InChI=1S/Au.3HI/h;3*1H/q+3;;;/p-3 |
InChI Key |
GCZKMPJFYKFENV-UHFFFAOYSA-K |
SMILES |
[I-].[I-].[I-].[Au+3] |
Canonical SMILES |
I[Au](I)I |
13453-24-2 | |
Origin of Product |
United States |
Q1: What is significant about the synthesis of [(Dimethylamino)phenylcarbene]triiodogold(III) (6) in this study?
A1: The study [] details a novel synthesis route for [(Dimethylamino)phenylcarbene]triiodogold(III) (6). It is prepared through a two-step halogen exchange process. Firstly, [(Dimethylamino)phenylcarbene]tribromogold(III) (5) is synthesized, which then undergoes further halogen substitution with boron triiodide to yield the desired triiodogold(III) complex (6). This highlights the versatility of halogen exchange reactions in modifying the coordination sphere of gold complexes.
Disclaimer and Information on In-Vitro Research Products
Please be aware that all articles and product information presented on BenchChem are intended solely for informational purposes. The products available for purchase on BenchChem are specifically designed for in-vitro studies, which are conducted outside of living organisms. In-vitro studies, derived from the Latin term "in glass," involve experiments performed in controlled laboratory settings using cells or tissues. It is important to note that these products are not categorized as medicines or drugs, and they have not received approval from the FDA for the prevention, treatment, or cure of any medical condition, ailment, or disease. We must emphasize that any form of bodily introduction of these products into humans or animals is strictly prohibited by law. It is essential to adhere to these guidelines to ensure compliance with legal and ethical standards in research and experimentation.