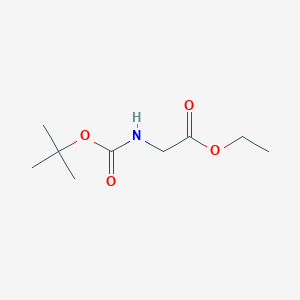
N-Boc-Glycine ethyl ester
Overview
Description
N-Boc-Glycine ethyl ester is an organic compound commonly used in synthetic organic chemistry. It is a derivative of glycine, where the amino group is protected by a tert-butoxycarbonyl (Boc) group. This compound is often utilized as an intermediate in the synthesis of various pharmaceuticals and biologically active molecules.
Preparation Methods
Synthetic Routes and Reaction Conditions
The synthesis of ethyl 2-((tert-butoxycarbonyl)amino)acetate typically involves the reaction of glycine ethyl ester with di-tert-butyl dicarbonate (Boc2O) in the presence of a base such as sodium hydroxide or 4-dimethylaminopyridine (DMAP). The reaction is usually carried out in an organic solvent like tetrahydrofuran (THF) or acetonitrile at ambient temperature .
Industrial Production Methods
For industrial production, the process is scaled up using similar reaction conditions but with optimized parameters to ensure high yield and purity. The use of continuous flow reactors has been explored to enhance the efficiency and sustainability of the production process .
Chemical Reactions Analysis
Types of Reactions
N-Boc-Glycine ethyl ester primarily undergoes substitution reactions due to the presence of the Boc-protected amino group. It can also participate in deprotection reactions where the Boc group is removed under acidic conditions.
Common Reagents and Conditions
Substitution Reactions: Common reagents include alkyl halides and acyl chlorides, typically in the presence of a base.
Deprotection Reactions: The Boc group can be removed using strong acids like trifluoroacetic acid (TFA) or hydrochloric acid (HCl) in organic solvents.
Major Products
The major products formed from these reactions include the deprotected amino acid ester and various substituted derivatives depending on the reagents used.
Scientific Research Applications
N-Boc-Glycine ethyl ester is widely used in scientific research, particularly in the fields of chemistry and biology. It serves as a key intermediate in the synthesis of peptides and other biologically active molecules. In medicinal chemistry, it is used to prepare various pharmaceutical compounds, including enzyme inhibitors and receptor agonists .
Mechanism of Action
The mechanism of action of ethyl 2-((tert-butoxycarbonyl)amino)acetate involves its role as a protected amino acid derivative. The Boc group protects the amino group during synthetic transformations, preventing unwanted side reactions. Upon deprotection, the free amino group can participate in further chemical reactions, such as peptide bond formation .
Comparison with Similar Compounds
Similar Compounds
Ethyl 2-((tert-butoxycarbonyl)amino)propanoate: Similar structure but with an additional methyl group.
Methyl 2-((tert-butoxycarbonyl)amino)acetate: Similar structure but with a methyl ester instead of an ethyl ester.
Uniqueness
N-Boc-Glycine ethyl ester is unique due to its specific combination of the Boc-protected amino group and the ethyl ester functionality, making it particularly useful in peptide synthesis and other applications where selective protection and deprotection of the amino group are required .
Properties
IUPAC Name |
ethyl 2-[(2-methylpropan-2-yl)oxycarbonylamino]acetate | |
---|---|---|
Source | PubChem | |
URL | https://pubchem.ncbi.nlm.nih.gov | |
Description | Data deposited in or computed by PubChem | |
InChI |
InChI=1S/C9H17NO4/c1-5-13-7(11)6-10-8(12)14-9(2,3)4/h5-6H2,1-4H3,(H,10,12) | |
Source | PubChem | |
URL | https://pubchem.ncbi.nlm.nih.gov | |
Description | Data deposited in or computed by PubChem | |
InChI Key |
CNIBHMMDDXGDNR-UHFFFAOYSA-N | |
Source | PubChem | |
URL | https://pubchem.ncbi.nlm.nih.gov | |
Description | Data deposited in or computed by PubChem | |
Canonical SMILES |
CCOC(=O)CNC(=O)OC(C)(C)C | |
Source | PubChem | |
URL | https://pubchem.ncbi.nlm.nih.gov | |
Description | Data deposited in or computed by PubChem | |
Molecular Formula |
C9H17NO4 | |
Source | PubChem | |
URL | https://pubchem.ncbi.nlm.nih.gov | |
Description | Data deposited in or computed by PubChem | |
Molecular Weight |
203.24 g/mol | |
Source | PubChem | |
URL | https://pubchem.ncbi.nlm.nih.gov | |
Description | Data deposited in or computed by PubChem | |
Synthesis routes and methods
Procedure details
Retrosynthesis Analysis
AI-Powered Synthesis Planning: Our tool employs the Template_relevance Pistachio, Template_relevance Bkms_metabolic, Template_relevance Pistachio_ringbreaker, Template_relevance Reaxys, Template_relevance Reaxys_biocatalysis model, leveraging a vast database of chemical reactions to predict feasible synthetic routes.
One-Step Synthesis Focus: Specifically designed for one-step synthesis, it provides concise and direct routes for your target compounds, streamlining the synthesis process.
Accurate Predictions: Utilizing the extensive PISTACHIO, BKMS_METABOLIC, PISTACHIO_RINGBREAKER, REAXYS, REAXYS_BIOCATALYSIS database, our tool offers high-accuracy predictions, reflecting the latest in chemical research and data.
Strategy Settings
Precursor scoring | Relevance Heuristic |
---|---|
Min. plausibility | 0.01 |
Model | Template_relevance |
Template Set | Pistachio/Bkms_metabolic/Pistachio_ringbreaker/Reaxys/Reaxys_biocatalysis |
Top-N result to add to graph | 6 |
Feasible Synthetic Routes
Q1: Why is N-Boc-Glycine ethyl ester used in the synthesis of Ledipasvir?
A1: this compound plays a vital role in constructing the complex structure of Ledipasvir. Specifically, it reacts with 1,1-dihalo-methyl cyclopropane under alkaline conditions to form a spiro compound. This spiro compound is a key intermediate that undergoes further transformations to ultimately yield the desired chiral intermediate of Ledipasvir [, ].
Q2: What are the advantages of this synthetic route using this compound?
A2: The research highlights several benefits of employing this specific synthetic pathway [, ]:
Disclaimer and Information on In-Vitro Research Products
Please be aware that all articles and product information presented on BenchChem are intended solely for informational purposes. The products available for purchase on BenchChem are specifically designed for in-vitro studies, which are conducted outside of living organisms. In-vitro studies, derived from the Latin term "in glass," involve experiments performed in controlled laboratory settings using cells or tissues. It is important to note that these products are not categorized as medicines or drugs, and they have not received approval from the FDA for the prevention, treatment, or cure of any medical condition, ailment, or disease. We must emphasize that any form of bodily introduction of these products into humans or animals is strictly prohibited by law. It is essential to adhere to these guidelines to ensure compliance with legal and ethical standards in research and experimentation.