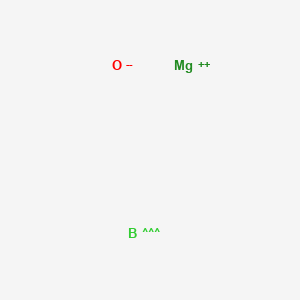
Magnesium hexaborate
Overview
Description
Magnesium hexaborate is an inorganic compound composed of magnesium, boron, and oxygen. It is known for its unique properties, including high thermal stability, mechanical strength, and resistance to corrosion. These characteristics make it valuable in various industrial and scientific applications.
Preparation Methods
Synthetic Routes and Reaction Conditions
Magnesium hexaborate can be synthesized through several methods. One common approach involves the reaction of magnesium oxide with boric acid under hydrothermal conditions. The reaction typically occurs at temperatures ranging from 120°C to 180°C, with a high B2O3/MgO molar ratio favoring the formation of this compound . Another method involves the precipitation of this compound from a supersaturated solution, followed by filtration and drying .
Industrial Production Methods
In industrial settings, this compound is often produced using a hydrothermal method. This involves mixing light-burned magnesia with boric acid and subjecting the mixture to high temperatures and pressures. The resulting product is then filtered, washed, and dried to obtain pure this compound .
Chemical Reactions Analysis
Types of Reactions
Magnesium hexaborate undergoes various chemical reactions, including:
Oxidation: this compound can be oxidized to form magnesium oxide and boron oxide.
Reduction: It can be reduced to elemental magnesium and boron under specific conditions.
Substitution: this compound can participate in substitution reactions where boron atoms are replaced by other elements.
Common Reagents and Conditions
Common reagents used in reactions with this compound include strong acids (e.g., hydrochloric acid) and bases (e.g., sodium hydroxide). The reactions typically occur under controlled temperatures and pressures to ensure the desired products are formed.
Major Products Formed
The major products formed from reactions involving this compound include magnesium oxide, boron oxide, and various substituted borates, depending on the specific reaction conditions and reagents used .
Scientific Research Applications
Magnesium hexaborate has a wide range of scientific research applications, including:
Chemistry: It is used as a catalyst in organic reactions and as a precursor for the synthesis of other borate compounds.
Biology: this compound is studied for its potential use in drug delivery systems and as an antibacterial agent.
Industry: This compound is used in the production of special glasses, ceramics, and as a flame retardant.
Mechanism of Action
The mechanism by which magnesium hexaborate exerts its effects involves its interaction with molecular targets and pathways. In biological systems, it can interact with cellular membranes, leading to structural damage and oxidative stress. This is particularly relevant in its antibacterial activity, where it disrupts the cell membrane of bacteria, leading to cell death .
Comparison with Similar Compounds
Magnesium hexaborate can be compared with other magnesium borates, such as magnesium tetraborate and magnesium diborate. While all these compounds share similar properties, this compound is unique due to its higher boron content, which imparts greater thermal stability and mechanical strength .
List of Similar Compounds
- Magnesium tetraborate
- Magnesium diborate
- Magnesium oxide
- Magnesium phosphate
This compound stands out among these compounds due to its specific structural and chemical properties, making it particularly valuable in high-temperature and high-strength applications.
Properties
InChI |
InChI=1S/B.Mg.O/q;+2;-2 | |
---|---|---|
Source | PubChem | |
URL | https://pubchem.ncbi.nlm.nih.gov | |
Description | Data deposited in or computed by PubChem | |
InChI Key |
YZUWTDQUEUJLAR-UHFFFAOYSA-N | |
Source | PubChem | |
URL | https://pubchem.ncbi.nlm.nih.gov | |
Description | Data deposited in or computed by PubChem | |
Canonical SMILES |
[B].[O-2].[Mg+2] | |
Source | PubChem | |
URL | https://pubchem.ncbi.nlm.nih.gov | |
Description | Data deposited in or computed by PubChem | |
Molecular Formula |
BMgO | |
Source | PubChem | |
URL | https://pubchem.ncbi.nlm.nih.gov | |
Description | Data deposited in or computed by PubChem | |
Related CAS |
12429-66-2 (MgB6O10), 13703-82-7 (HBO2.1/2Mg) | |
Record name | Magnesium borate | |
Source | ChemIDplus | |
URL | https://pubchem.ncbi.nlm.nih.gov/substance/?source=chemidplus&sourceid=0012007624 | |
Description | ChemIDplus is a free, web search system that provides access to the structure and nomenclature authority files used for the identification of chemical substances cited in National Library of Medicine (NLM) databases, including the TOXNET system. | |
Molecular Weight |
51.12 g/mol | |
Source | PubChem | |
URL | https://pubchem.ncbi.nlm.nih.gov | |
Description | Data deposited in or computed by PubChem | |
CAS No. |
12007-62-4 | |
Record name | Magnesium borate | |
Source | ChemIDplus | |
URL | https://pubchem.ncbi.nlm.nih.gov/substance/?source=chemidplus&sourceid=0012007624 | |
Description | ChemIDplus is a free, web search system that provides access to the structure and nomenclature authority files used for the identification of chemical substances cited in National Library of Medicine (NLM) databases, including the TOXNET system. | |
Synthesis routes and methods I
Procedure details
Synthesis routes and methods II
Procedure details
Disclaimer and Information on In-Vitro Research Products
Please be aware that all articles and product information presented on BenchChem are intended solely for informational purposes. The products available for purchase on BenchChem are specifically designed for in-vitro studies, which are conducted outside of living organisms. In-vitro studies, derived from the Latin term "in glass," involve experiments performed in controlled laboratory settings using cells or tissues. It is important to note that these products are not categorized as medicines or drugs, and they have not received approval from the FDA for the prevention, treatment, or cure of any medical condition, ailment, or disease. We must emphasize that any form of bodily introduction of these products into humans or animals is strictly prohibited by law. It is essential to adhere to these guidelines to ensure compliance with legal and ethical standards in research and experimentation.