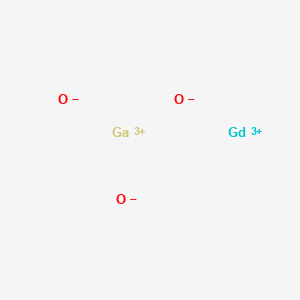
Gadolinium gallium trioxide
Overview
Description
Gadolinium gallium trioxide is an inorganic compound composed of gadolinium, gallium, and oxygen It is known for its unique properties, including high thermal stability and magnetic susceptibility
Mechanism of Action
Target of Action
Gadolinium gallium trioxide, also known as Gadolinium gallium garnet (GGG), is a synthetic crystalline material . It primarily targets the magneto-optical films and is used as a substrate material for these films . It also finds use in jewelry as a diamond simulant .
Mode of Action
The interaction of GGG with its targets is primarily through its excellent mechanical, thermal, and optical properties . For instance, in the case of magneto-optical films, GGG provides a stable and high-quality substrate that allows for the growth and performance of these films . The cubic lattice structure, density, and hardness of GGG play a crucial role in its interaction with its targets .
Biochemical Pathways
It’s worth noting that gadolinium-based contrast agents (gbcas), which contain gadolinium, have been associated with gadolinium release and tissue deposition that may cause short- and long-term toxicity in several organs .
Pharmacokinetics
In the context of its use, ggg exhibits high thermal conductivity, a high melting point, and is typically insoluble in water .
Result of Action
The primary result of GGG’s action is the enhancement of the performance of magneto-optical films. It provides a high-quality substrate that allows for the growth and performance of these films . In addition, GGG’s hardness and density make it suitable for use as a diamond simulant in jewelry .
Action Environment
The action, efficacy, and stability of GGG can be influenced by environmental factors such as temperature and pressure. For instance, the phase change from cubic to monoclinic structure in GGG takes place at 1200 °C . Furthermore, the addition of Gd2O3 particles to the pure aluminum anode has been shown to improve its corrosion resistance, making the discharge stable and uniform, which is more suitable as an anode material for aluminum-air batteries .
Biochemical Analysis
Biochemical Properties
It has been used in the production of batteries, where it has shown to improve the corrosion resistance of the anode material . This suggests that Gadolinium gallium trioxide may interact with certain metal ions and influence electrochemical reactions.
Molecular Mechanism
It has been suggested that the compound may affect charge transfer on the anode surface in batteries . This indicates that this compound may interact with certain biomolecules and influence their activity.
Temporal Effects in Laboratory Settings
It has been reported that the addition of this compound to pure aluminum has a positive effect on the performance of the battery anode material .
Preparation Methods
Synthetic Routes and Reaction Conditions: Gadolinium gallium trioxide can be synthesized using several methods, including solid-phase reaction synthesis, sol-gel method, and chemical co-precipitation. The solid-phase reaction synthesis involves mixing gadolinium oxide and gallium oxide powders, followed by high-temperature calcination. The sol-gel method uses metal alkoxides or nitrates as precursors, which are hydrolyzed and condensed to form a gel. This gel is then dried and calcined to obtain the final product. The chemical co-precipitation method involves precipitating gadolinium and gallium hydroxides from their respective salt solutions, followed by thermal decomposition to form the oxide.
Industrial Production Methods: In industrial settings, this compound is typically produced using the solid-phase reaction synthesis due to its simplicity and scalability. The process involves mixing stoichiometric amounts of gadolinium oxide and gallium oxide, followed by calcination at temperatures ranging from 1000°C to 1500°C. This method ensures the formation of a homogeneous product with high purity.
Chemical Reactions Analysis
Types of Reactions: Gadolinium gallium trioxide undergoes various chemical reactions, including oxidation, reduction, and substitution. It is relatively stable under normal conditions but can react with strong acids and bases.
Common Reagents and Conditions:
Oxidation: this compound can be oxidized in the presence of strong oxidizing agents such as hydrogen peroxide or potassium permanganate.
Reduction: It can be reduced using strong reducing agents like hydrogen gas or sodium borohydride.
Substitution: Substitution reactions can occur when gadolinium or gallium atoms are replaced by other metal ions in the lattice structure.
Major Products Formed: The major products formed from these reactions depend on the specific reagents and conditions used. For example, oxidation may result in the formation of higher oxides, while reduction can lead to the formation of lower oxides or elemental metals.
Scientific Research Applications
Gadolinium gallium trioxide has a wide range of scientific research applications:
Chemistry: It is used as a catalyst in various chemical reactions, including oxidation and hydrogenation processes.
Biology: Gadolinium-based compounds are used as contrast agents in magnetic resonance imaging (MRI) due to their paramagnetic properties.
Medicine: Research is ongoing into the use of this compound in targeted drug delivery systems and cancer treatment.
Industry: It is used in the production of high-performance ceramics, phosphors for lighting and display technologies, and as a component in solid-state lasers.
Comparison with Similar Compounds
Gadolinium(III) oxide: Similar in composition but lacks the gallium component, making it less versatile in certain applications.
Gallium(III) oxide: Known for its semiconductor properties, but does not possess the magnetic properties of gadolinium gallium trioxide.
Europium(III) oxide: Another rare-earth oxide with applications in phosphors and electronics, but with different electronic and magnetic properties.
Uniqueness: this compound is unique due to its combination of magnetic, electronic, and thermal properties. This makes it suitable for a wide range of applications, from medical imaging to advanced materials science.
Properties
IUPAC Name |
gallium;gadolinium(3+);oxygen(2-) | |
---|---|---|
Source | PubChem | |
URL | https://pubchem.ncbi.nlm.nih.gov | |
Description | Data deposited in or computed by PubChem | |
InChI |
InChI=1S/Ga.Gd.3O/q2*+3;3*-2 | |
Source | PubChem | |
URL | https://pubchem.ncbi.nlm.nih.gov | |
Description | Data deposited in or computed by PubChem | |
InChI Key |
ZPDRQAVGXHVGTB-UHFFFAOYSA-N | |
Source | PubChem | |
URL | https://pubchem.ncbi.nlm.nih.gov | |
Description | Data deposited in or computed by PubChem | |
Canonical SMILES |
[O-2].[O-2].[O-2].[Ga+3].[Gd+3] | |
Source | PubChem | |
URL | https://pubchem.ncbi.nlm.nih.gov | |
Description | Data deposited in or computed by PubChem | |
Molecular Formula |
GaGdO3 | |
Source | PubChem | |
URL | https://pubchem.ncbi.nlm.nih.gov | |
Description | Data deposited in or computed by PubChem | |
DSSTOX Substance ID |
DTXSID00923503 | |
Record name | Gadolinium gallium oxide (1/1/3) | |
Source | EPA DSSTox | |
URL | https://comptox.epa.gov/dashboard/DTXSID00923503 | |
Description | DSSTox provides a high quality public chemistry resource for supporting improved predictive toxicology. | |
Molecular Weight |
275.0 g/mol | |
Source | PubChem | |
URL | https://pubchem.ncbi.nlm.nih.gov | |
Description | Data deposited in or computed by PubChem | |
CAS No. |
12024-36-1, 12063-81-9 | |
Record name | Gadolinium gallium oxide (Gd3Ga5O12) | |
Source | ChemIDplus | |
URL | https://pubchem.ncbi.nlm.nih.gov/substance/?source=chemidplus&sourceid=0012024361 | |
Description | ChemIDplus is a free, web search system that provides access to the structure and nomenclature authority files used for the identification of chemical substances cited in National Library of Medicine (NLM) databases, including the TOXNET system. | |
Record name | Gadolinium gallium trioxide | |
Source | ChemIDplus | |
URL | https://pubchem.ncbi.nlm.nih.gov/substance/?source=chemidplus&sourceid=0012063819 | |
Description | ChemIDplus is a free, web search system that provides access to the structure and nomenclature authority files used for the identification of chemical substances cited in National Library of Medicine (NLM) databases, including the TOXNET system. | |
Record name | Gadolinium gallium oxide (1/1/3) | |
Source | EPA DSSTox | |
URL | https://comptox.epa.gov/dashboard/DTXSID00923503 | |
Description | DSSTox provides a high quality public chemistry resource for supporting improved predictive toxicology. | |
Record name | Gadolinium gallium trioxide | |
Source | European Chemicals Agency (ECHA) | |
URL | https://echa.europa.eu/substance-information/-/substanceinfo/100.031.856 | |
Description | The European Chemicals Agency (ECHA) is an agency of the European Union which is the driving force among regulatory authorities in implementing the EU's groundbreaking chemicals legislation for the benefit of human health and the environment as well as for innovation and competitiveness. | |
Explanation | Use of the information, documents and data from the ECHA website is subject to the terms and conditions of this Legal Notice, and subject to other binding limitations provided for under applicable law, the information, documents and data made available on the ECHA website may be reproduced, distributed and/or used, totally or in part, for non-commercial purposes provided that ECHA is acknowledged as the source: "Source: European Chemicals Agency, http://echa.europa.eu/". Such acknowledgement must be included in each copy of the material. ECHA permits and encourages organisations and individuals to create links to the ECHA website under the following cumulative conditions: Links can only be made to webpages that provide a link to the Legal Notice page. | |
Disclaimer and Information on In-Vitro Research Products
Please be aware that all articles and product information presented on BenchChem are intended solely for informational purposes. The products available for purchase on BenchChem are specifically designed for in-vitro studies, which are conducted outside of living organisms. In-vitro studies, derived from the Latin term "in glass," involve experiments performed in controlled laboratory settings using cells or tissues. It is important to note that these products are not categorized as medicines or drugs, and they have not received approval from the FDA for the prevention, treatment, or cure of any medical condition, ailment, or disease. We must emphasize that any form of bodily introduction of these products into humans or animals is strictly prohibited by law. It is essential to adhere to these guidelines to ensure compliance with legal and ethical standards in research and experimentation.