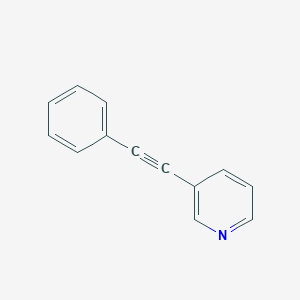
3-Phenylethynyl-pyridine
Overview
Description
3-(Phenylethynyl)pyridine is an organic compound that belongs to the class of pyridines, which are heterocyclic aromatic organic compounds This compound is characterized by a phenylethynyl group attached to the third position of the pyridine ring
Mechanism of Action
Target of Action
3-Phenylethynyl-pyridine primarily targets the Src kinase , a non-receptor tyrosine kinase that plays a crucial role in cell proliferation, differentiation, survival, and migration . It also interacts with the metabotropic glutamate receptor 5 (mGluR5) , which is involved in various physiological and pathological processes, including learning, memory, anxiety, and the pathophysiology of fragile X syndrome .
Mode of Action
This compound acts as an antagonist at the mGluR5 receptor . It inhibits the receptor’s activity, thereby modulating the downstream signaling pathways . The compound also inhibits Src kinase, disrupting its role in various cellular processes .
Biochemical Pathways
The inhibition of Src kinase by this compound affects various biochemical pathways. Src kinase is involved in multiple signaling pathways, including those related to cell growth and survival . By inhibiting Src kinase, this compound can potentially disrupt these pathways, leading to antiproliferative effects .
Pharmacokinetics
The compound’s effectiveness as an inhibitor suggests it has sufficient bioavailability to interact with its targets .
Result of Action
The inhibition of Src kinase and mGluR5 by this compound can lead to various cellular effects. For instance, it can induce antiproliferative effects in cancer cells . Additionally, the compound’s action on mGluR5 can influence neuronal activity, potentially impacting processes such as learning and memory .
Action Environment
The action of this compound can be influenced by various environmental factors. For example, the presence of other molecules that bind to the same targets can affect the compound’s efficacy. Additionally, factors such as pH and temperature can potentially influence the stability and activity of the compound .
Biochemical Analysis
Biochemical Properties
3-Phenylethynyl-pyridine has been identified as a potential inhibitor of Src, a non-receptor tyrosine kinase . It interacts with Src, affecting its activity and potentially influencing biochemical reactions .
Cellular Effects
The effects of this compound on cells are largely tied to its interaction with Src. As Src is involved in various cellular processes, including cell signaling pathways, gene expression, and cellular metabolism, the impact of this compound is significant .
Molecular Mechanism
This compound exerts its effects at the molecular level through its interaction with Src. It may bind to Src, inhibiting or activating it, and causing changes in gene expression .
Temporal Effects in Laboratory Settings
Its interaction with Src suggests that it may have long-term effects on cellular function .
Preparation Methods
Synthetic Routes and Reaction Conditions
The synthesis of 3-(Phenylethynyl)pyridine typically involves the coupling of a phenylacetylene with a pyridine derivative. One common method is the Sonogashira coupling reaction, which involves the reaction of a halogenated pyridine with phenylacetylene in the presence of a palladium catalyst and a copper co-catalyst . The reaction is usually carried out under mild conditions, making it a convenient method for the synthesis of this compound.
Industrial Production Methods
While specific industrial production methods for 3-(Phenylethynyl)pyridine are not extensively documented, the principles of large-scale organic synthesis can be applied. This includes optimizing reaction conditions to maximize yield and purity, using cost-effective reagents, and employing efficient purification techniques such as distillation or chromatography.
Chemical Reactions Analysis
Types of Reactions
3-(Phenylethynyl)pyridine can undergo various types of chemical reactions, including:
Oxidation: This compound can be oxidized to form corresponding pyridine N-oxides.
Reduction: Reduction reactions can convert the triple bond in the phenylethynyl group to a double or single bond.
Substitution: The phenylethynyl group can be substituted with other functional groups through reactions such as halogenation or nitration.
Common Reagents and Conditions
Oxidation: Common oxidizing agents include hydrogen peroxide and peracids.
Reduction: Catalytic hydrogenation using palladium or platinum catalysts is often employed.
Substitution: Halogenation can be achieved using halogens like chlorine or bromine in the presence of a catalyst.
Major Products
The major products formed from these reactions depend on the specific conditions and reagents used. For example, oxidation can yield pyridine N-oxides, while reduction can produce phenylethenyl or phenylethyl derivatives.
Scientific Research Applications
3-(Phenylethynyl)pyridine has a wide range of applications in scientific research:
Chemistry: It is used as a building block in the synthesis of more complex organic molecules.
Medicine: Research has explored its potential as a therapeutic agent due to its ability to interact with specific molecular targets.
Industry: It is used in the development of advanced materials, including polymers and electronic devices.
Comparison with Similar Compounds
Similar Compounds
- 2-(Phenylethynyl)pyridine
- 4-(Phenylethynyl)pyridine
- 3-(Phenylethynyl)quinoline
Uniqueness
3-(Phenylethynyl)pyridine is unique due to its specific substitution pattern, which imparts distinct chemical and biological properties. Compared to its isomers, it may exhibit different reactivity and selectivity in chemical reactions, as well as varying biological activities.
Properties
IUPAC Name |
3-(2-phenylethynyl)pyridine | |
---|---|---|
Source | PubChem | |
URL | https://pubchem.ncbi.nlm.nih.gov | |
Description | Data deposited in or computed by PubChem | |
InChI |
InChI=1S/C13H9N/c1-2-5-12(6-3-1)8-9-13-7-4-10-14-11-13/h1-7,10-11H | |
Source | PubChem | |
URL | https://pubchem.ncbi.nlm.nih.gov | |
Description | Data deposited in or computed by PubChem | |
InChI Key |
ZLYFXWKSLUXFMU-UHFFFAOYSA-N | |
Source | PubChem | |
URL | https://pubchem.ncbi.nlm.nih.gov | |
Description | Data deposited in or computed by PubChem | |
Canonical SMILES |
C1=CC=C(C=C1)C#CC2=CN=CC=C2 | |
Source | PubChem | |
URL | https://pubchem.ncbi.nlm.nih.gov | |
Description | Data deposited in or computed by PubChem | |
Molecular Formula |
C13H9N | |
Source | PubChem | |
URL | https://pubchem.ncbi.nlm.nih.gov | |
Description | Data deposited in or computed by PubChem | |
DSSTOX Substance ID |
DTXSID10449188 | |
Record name | 3-Phenylethynyl-pyridine | |
Source | EPA DSSTox | |
URL | https://comptox.epa.gov/dashboard/DTXSID10449188 | |
Description | DSSTox provides a high quality public chemistry resource for supporting improved predictive toxicology. | |
Molecular Weight |
179.22 g/mol | |
Source | PubChem | |
URL | https://pubchem.ncbi.nlm.nih.gov | |
Description | Data deposited in or computed by PubChem | |
CAS No. |
13238-38-5 | |
Record name | 3-Phenylethynyl-pyridine | |
Source | EPA DSSTox | |
URL | https://comptox.epa.gov/dashboard/DTXSID10449188 | |
Description | DSSTox provides a high quality public chemistry resource for supporting improved predictive toxicology. | |
Synthesis routes and methods I
Procedure details
Synthesis routes and methods II
Procedure details
Retrosynthesis Analysis
AI-Powered Synthesis Planning: Our tool employs the Template_relevance Pistachio, Template_relevance Bkms_metabolic, Template_relevance Pistachio_ringbreaker, Template_relevance Reaxys, Template_relevance Reaxys_biocatalysis model, leveraging a vast database of chemical reactions to predict feasible synthetic routes.
One-Step Synthesis Focus: Specifically designed for one-step synthesis, it provides concise and direct routes for your target compounds, streamlining the synthesis process.
Accurate Predictions: Utilizing the extensive PISTACHIO, BKMS_METABOLIC, PISTACHIO_RINGBREAKER, REAXYS, REAXYS_BIOCATALYSIS database, our tool offers high-accuracy predictions, reflecting the latest in chemical research and data.
Strategy Settings
Precursor scoring | Relevance Heuristic |
---|---|
Min. plausibility | 0.01 |
Model | Template_relevance |
Template Set | Pistachio/Bkms_metabolic/Pistachio_ringbreaker/Reaxys/Reaxys_biocatalysis |
Top-N result to add to graph | 6 |
Feasible Synthetic Routes
Disclaimer and Information on In-Vitro Research Products
Please be aware that all articles and product information presented on BenchChem are intended solely for informational purposes. The products available for purchase on BenchChem are specifically designed for in-vitro studies, which are conducted outside of living organisms. In-vitro studies, derived from the Latin term "in glass," involve experiments performed in controlled laboratory settings using cells or tissues. It is important to note that these products are not categorized as medicines or drugs, and they have not received approval from the FDA for the prevention, treatment, or cure of any medical condition, ailment, or disease. We must emphasize that any form of bodily introduction of these products into humans or animals is strictly prohibited by law. It is essential to adhere to these guidelines to ensure compliance with legal and ethical standards in research and experimentation.