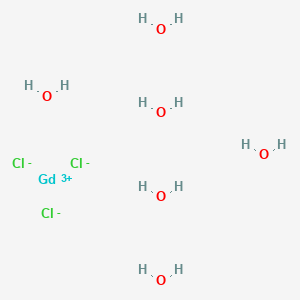
Gadolinium(III) chloride hexahydrate
Overview
Description
Gadolinium(III) chloride hexahydrate (GdCl₃·6H₂O) is a hygroscopic, water-soluble lanthanide compound with the chemical formula Cl₃Gd·6H₂O and a molecular weight of ~371.69 g/mol. It exhibits strong ionic interactions and coordination chemistry due to its six water molecules, which facilitate diverse complex formations . The gadolinium ion (Gd³⁺) has a high magnetic moment (7.94 µB), making it valuable in magnetic resonance imaging (MRI) contrast agents, upconversion nanoparticles (UCNPs), and catalysis . Its crystalline structure contributes to distinct thermal and optical behaviors, with applications spanning electronics, phosphors, laser media, and biomedical research . Commercial grades typically range from 99% to 99.99% purity, depending on synthetic protocols .
Preparation Methods
Direct Synthesis from Gadolinium Oxide and Hydrochloric Acid
The most widely documented method for synthesizing GdCl₃·6H₂O involves the reaction of gadolinium(III) oxide (Gd₂O₃) with concentrated hydrochloric acid (HCl). This exothermic process proceeds according to the equation:
2\text{O}3 + 6\text{HCl} + 9\text{H}2\text{O} \rightarrow 2\text{GdCl}3\cdot6\text{H}2\text{O} + 3\text{H}2\text{O}
In practice, Gd₂O₃ of at least 99.9% purity is dissolved in a stoichiometric excess of HCl (37% w/w) under reflux at 80–90°C for 12–24 hours . The hexahydrate crystallizes upon slow evaporation at room temperature, yielding colorless hygroscopic crystals. Gravimetric analysis of the product typically confirms a chloride-to-gadolinium molar ratio of 3:1, with residual water content verified by Karl Fischer titration .
Optimization of Reaction Parameters
-
Acid Concentration : A 6:1 molar ratio of HCl to Gd₂O₃ ensures complete dissolution while minimizing residual Cl⁻. Excess HCl increases the risk of forming oxychloride impurities (e.g., GdOCl) .
-
Temperature Control : Maintaining temperatures below 100°C prevents partial dehydration to lower hydrates (e.g., GdCl₃·3H₂O) .
-
Crystallization Conditions : Slow evaporation in a desiccator with saturated NaCl solution (33% relative humidity) favors hexahydrate formation over lower hydrates .
Metathesis Reactions Using Ammonium Chloride
An alternative route employs ammonium chloride (NH₄Cl) as a chlorinating agent for Gd₂O₃. This solid-state reaction avoids aqueous conditions, reducing hydrolysis risks:
2\text{O}3 + 6\text{NH}4\text{Cl} \rightarrow 2\text{GdCl}3 + 3\text{H}2\text{O} + 6\text{NH}3\uparrow
The process involves grinding stoichiometric amounts of Gd₂O₃ and NH₄Cl, followed by heating at 200–250°C under dynamic vacuum . Sublimation of NH₃ drives the reaction to completion within 8–12 hours. The resultant anhydrous GdCl₃ is hygroscopic and readily absorbs atmospheric moisture to form the hexahydrate.
Advantages and Limitations
-
Purity : Anhydrous GdCl₃ produced via this method exhibits ≤0.1% oxide impurities, as confirmed by X-ray diffraction .
-
Scalability : Large-scale synthesis is feasible but requires stringent moisture control during hydration to prevent uneven water uptake .
Solvent-Mediated Recrystallization Techniques
Recrystallization from polar aprotic solvents is critical for removing ionic impurities (e.g., GdOCl, NH₄⁺). Methanol, ethanol, and 2-propanol are commonly used due to their moderate solubility parameters (Table 1).
Table 1: Solubility of GdCl₃·6H₂O in Organic Solvents at 298.2 K
Solvent | Solubility (mol/kg) | Solid Phase Identified |
---|---|---|
Methanol | 4.21 | GdCl₃·4CH₃OH |
Ethanol | 2.43 | GdCl₃·4.1C₂H₅OH |
2-Propanol | 0.32 | GdCl₃·3.0–3.1C₃H₇OH |
Tetrahydrofuran | 0.0725 | GdCl₃·3THF |
Data sourced from equilibrium studies using isothermal saturation methods .
Protocol for Methanol Recrystallization
-
Dissolve crude GdCl₃·6H₂O in anhydrous methanol (5:1 v/w) at 50°C.
-
Filter through a 0.2 μm PTFE membrane to remove insoluble oxides.
-
Cool to −20°C to precipitate GdCl₃·4CH₃OH.
-
Desolvate under vacuum (10⁻³ mbar, 80°C) to regenerate the hexahydrate .
This method reduces NH₄⁺ contamination by 90% compared to direct aqueous synthesis .
Analysis of Impurities and Purification Methods
Common impurities in GdCl₃·6H₂O include residual NH₄⁺, GdOCl, and solvent adducts. Mitigation strategies include:
-
Ion Exchange Chromatography : Passing acidic solutions through Dowex 50WX8 resin removes NH₄⁺ with >99% efficiency .
-
Selective Precipitation : Adjusting pH to 4.5–5.0 with dilute NH₄OH precipitates GdOCl, leaving Gd³⁺ in solution .
Comparative Evaluation of Synthesis Methods
Table 2: Performance Metrics of GdCl₃·6H₂O Synthesis Routes
Method | Yield (%) | Purity (%) | Key Impurities |
---|---|---|---|
HCl Dissolution | 92–95 | 99.5 | Cl⁻, H₂O |
NH₄Cl Metathesis | 85–88 | 99.9 | NH₄⁺, GdOCl |
Methanol Recrystallization | 78–82 | 99.99 | Solvent residues |
Scientific Research Applications
Medical Applications
Magnetic Resonance Imaging (MRI)
Gadolinium(III) chloride hexahydrate is primarily used in the medical field as a contrast agent for MRI. Gadolinium ions (Gd³⁺) are paramagnetic, which enhances the relaxation times of nearby protons in the body, resulting in improved image quality. However, GdCl₃·6H₂O itself cannot be used directly due to its low solubility at physiological pH and potential toxicity when "free" gadolinium is present in the body. Therefore, it is typically utilized in chelated forms with ligands like diethylenetriaminepentaacetic acid (DTPA) to ensure safety and efficacy .
Case Study: Gadolinium-based Contrast Agents
A study published in Radiology demonstrated that gadolinium-based contrast agents significantly improve the detection of tumors compared to non-contrast MRI scans. The use of GdCl₃·6H₂O as a precursor for these agents has been pivotal in enhancing diagnostic imaging techniques .
Nanoparticle Synthesis
GdCl₃·6H₂O is utilized as a precursor for synthesizing gadolinium oxide nanoparticles, which have applications in drug delivery and targeted therapy due to their biocompatibility and magnetic properties. The nanoparticles can be engineered to carry therapeutic agents directly to cancer cells, enhancing treatment efficacy while minimizing side effects .
Case Study: Gadolinium Oxide Nanoparticles
Research published in Nanomedicine highlighted the effectiveness of gadolinium oxide nanoparticles synthesized from GdCl₃·6H₂O for targeted drug delivery systems. The study showed that these nanoparticles could effectively deliver chemotherapeutic agents to tumor sites while avoiding healthy tissues, significantly improving treatment outcomes .
Other Notable Applications
Microwave Applications
this compound has been explored for microwave applications due to its dielectric properties. It can be used in the development of materials that require specific electromagnetic characteristics .
Mechanism of Action
The compound exerts its effects primarily through its paramagnetic properties. In MRI, gadolinium(III) ions enhance the contrast of images by shortening the relaxation times of protons in water molecules within the body. This is achieved through the interaction of the unpaired electrons in gadolinium with the magnetic field, which influences the relaxation processes of nearby hydrogen nuclei .
Comparison with Similar Compounds
Lanthanide Chloride Hexahydrates
Lanthanide chlorides share similar hydration states and coordination properties but differ in ionic radii, magnetic moments, and applications (Table 1).
Table 1: Comparison of Lanthanide Chloride Hexahydrates
Compound | Molecular Weight (g/mol) | Magnetic Moment (µB) | Key Applications | Purity (%) |
---|---|---|---|---|
GdCl₃·6H₂O | 371.69 | 7.94 | MRI contrast agents, UCNPs, catalysts | 99.9–99.99 |
TbCl₃·6H₂O | 373.38 | 9.72 | Green phosphors, magneto-optical devices | 99.9 |
DyCl₃·6H₂O | 378.90 | 10.64 | High-strength magnets, neutron absorbers | 99.9 |
YCl₃·6H₂O | 303.26 | 0 (Y³⁺ non-magnetic) | UCNP matrices, laser crystals | 99.99 |
LuCl₃·6H₂O | 389.37 | 0 (Lu³⁺ non-magnetic) | Scintillators, nuclear medicine | 99.9 |
Key Differences:
- Magnetic Properties: Gd³⁺ has the highest spin-only magnetic moment among non-radioactive lanthanides, making it ideal for MRI. In contrast, Dy³⁺ and Tb³⁺ exhibit higher moments but are less biocompatible .
- Coordination Chemistry: Smaller lanthanides (e.g., Lu³⁺) form tighter coordination spheres, affecting extraction efficiency. For example, Gd³⁺ is more efficiently separated using P507 resin than Tb³⁺ or Dy³⁺ due to ionic radius differences .
- Applications: YCl₃·6H₂O lacks magnetic utility but serves as a UCNP host due to its optical transparency, while GdCl₃·6H₂O enhances relaxivity in hybrid nanomaterials .
Nitrate Counterparts: Gd(NO₃)₃·6H₂O vs. GdCl₃·6H₂O
Gadolinium nitrate hexahydrate (Gd(NO₃)₃·6H₂O) is another common precursor with distinct reactivity:
- Solubility: Nitrates are more soluble in polar organic solvents (e.g., ethanol), whereas chlorides prefer aqueous media .
- Oxidizing Nature: Nitrates decompose exothermically, limiting their use in high-temperature syntheses. Chlorides are thermally stable up to 100°C .
- Biological Use: GdCl₃·6H₂O suppresses Kupffer cells in liver studies, while Gd(NO₃)₃·6H₂O is employed in anti-inflammatory drug formulations .
Non-Lanthanide Hexahydrates
Iron(III) Chloride Hexahydrate (FeCl₃·6H₂O):
- Applications: Water treatment, etching, and catalysis.
- Differences: Fe³⁺ has a lower magnetic moment (5.92 µB) and forms acidic solutions, unlike neutral GdCl₃·6H₂O .
Aluminum(III) Chloride Hexahydrate (AlCl₃·6H₂O):
- Applications: Friedel-Crafts catalyst, pesticide production.
- Differences: Al³⁺ has a smaller ionic radius (0.535 Å vs. Gd³⁺: 0.938 Å), leading to weaker coordination with water .
Nanotechnology and UCNPs
GdCl₃·6H₂O is doped into NaYF₄ UCNPs to enhance upconversion luminescence and MRI contrast. At 20% Gd³⁺ doping, nanoparticles exhibit optimal luminescence intensity and minimal aging effects . In contrast, YbCl₃·6H₂O-doped UCNPs prioritize near-infrared emission but lack magnetic functionality .
Catalysis
GdCl₃·6H₂O catalyzes α-amino nitrile synthesis with 85% yield, outperforming La(NO₃)₃·6H₂O (70%) due to stronger Lewis acidity . YbCl₃·6H₂O, however, excels in aldol reactions owing to its smaller ionic radius .
Biological Activity
Gadolinium(III) chloride hexahydrate (GdCl₃·6H₂O) is a compound of significant interest in various fields, including medicine, toxicology, and materials science. This article reviews the biological activity of GdCl₃·6H₂O, focusing on its toxicity, potential therapeutic uses, and mechanisms of action.
This compound is a colorless, hygroscopic solid that is soluble in water. It has a molecular weight of approximately 371.69 g/mol and is characterized by its high magnetic properties due to the presence of unpaired electrons in the gadolinium ion (Gd³⁺) . The compound can be synthesized from gadolinium oxide or gadolinium chloride in concentrated hydrochloric acid followed by evaporation .
In Vitro Studies
Recent studies have evaluated the cytotoxic effects of GdCl₃·6H₂O on various cell lines. An in vitro study using V79-4 cells demonstrated that GdCl₃ did not induce significant cytotoxicity but exhibited clastogenic effects at concentrations above 0.25 mM . The findings indicated:
- Cell Viability : Significant reduction at 1.0 mM after 24 hours.
- DNA Damage : No significant DNA damage detected.
- Clastogenic Potential : Induction of chromosomal damage was observed, particularly at higher concentrations.
In Vivo Studies
In vivo assessments have shown varying degrees of toxicity associated with GdCl₃·6H₂O. A notable study involved administering the compound to Wistar rats over 28 days. Key findings included:
- Dosage Levels : Rats were exposed to doses of 0, 40, 200, or 1,000 mg/kg-day.
- Observations : At the highest dose (423 mg Gd/kg-day), lesions were noted in the stomach and significant changes in serum chemistry were observed.
- Toxicity Levels : The no-observed-adverse-effect level (NOAEL) was determined to be 84.7 mg/kg-day, while the lowest-observed-adverse-effect level (LOAEL) was established at 423 mg/kg-day based on observed lesions .
Gadolinium compounds are known for their effects on macrophages and other immune cells. GdCl₃·6H₂O has been identified as a macrophage inhibitor, specifically targeting Kupffer cells in the liver. This inhibition can modulate immune responses and has implications for inflammatory diseases .
Therapeutic Applications
The unique properties of gadolinium compounds have led to their use in medical imaging, particularly in magnetic resonance imaging (MRI). The high magnetic susceptibility of Gd³⁺ ions enhances contrast during imaging procedures. Furthermore, ongoing research explores the potential for GdCl₃·6H₂O in drug delivery systems due to its ability to form complexes with various biomolecules.
Case Study 1: Clastogenic Effects in Cell Lines
A study published in June 2020 assessed the clastogenic potential of GdCl₃·6H₂O on V79-4 cells. The results indicated a significant increase in micronucleus formation at concentrations as low as 0.25 mM, confirming its potential as a clastogen despite low cytotoxicity .
Case Study 2: Subchronic Toxicity in Rats
In a subchronic toxicity study involving dietary exposure to GdCl₃·6H₂O over 12 weeks, researchers observed no adverse effects on growth or blood parameters at lower doses. However, higher doses resulted in histological changes and biochemical alterations indicative of toxicity .
Summary Table of Toxicity Data
Study Type | Organism/Cell Line | Dose Range (mg/kg-day) | Key Findings |
---|---|---|---|
In Vitro | V79-4 cells | 0.25 - 1.0 mM | Clastogenic effects observed; no significant cytotoxicity |
In Vivo | Wistar rats | 0 - 1000 | NOAEL: 84.7 mg/kg-day; LOAEL: 423 mg/kg-day; stomach lesions observed |
Chemical Reactions Analysis
Complexation with Chelating Agents
GdCl₃·6H₂O reacts with polydentate ligands to form stable MRI contrast agents:
Reaction with DTPA (diethylenetriaminepentaacetic acid) :
The product, gadopentetate dimeglumine, has a coordination number of 9 (six from DTPA, one from H₂O) .
Property | Value |
---|---|
Coordination geometry | Distorted tricapped trigonal prism |
Magnetic moment (μeff) | 7.94 μB (theoretical) |
Water exchange rate (kₑˣ) | ~3.3 × 10⁶ s⁻¹ |
Stability constant (log K) | 22.5–23.1 |
This complex enhances MRI contrast by accelerating proton relaxation in water .
Solubility and Adduct Formation
GdCl₃·6H₂O exhibits unique solubility behavior in organic solvents, forming ethanolates and iso-propanolates:
Ethanol adduct :
Equilibrium studies in ethanol show a solubility of 2.43 mol/kg at 25°C .
Iso-propanol adduct :
These adducts are characterized by NMR and X-ray diffraction .
Redox Reactions
Gd³⁺ is redox-inert under physiological conditions, but reacts with strong reducing agents:
Reduction with lithium aluminum hydride :
This reaction produces gadolinium hydride, a potential hydrogen storage material .
Biological Interactions
GdCl₃·6H₂O selectively inhibits Kupffer cells (liver macrophages):
Q & A
Q. Basic: What are the critical handling and storage protocols for GdCl₃·6H₂O in laboratory settings?
GdCl₃·6H₂O is highly hygroscopic, requiring strict moisture control. Store in airtight containers under inert gas (e.g., argon) within desiccators containing anhydrous calcium sulfate or silica gel. Conduct weighing and synthesis in glove boxes with <5% humidity to prevent unintended hydration or decomposition . For long-term storage, maintain temperatures below 25°C in dark, dry environments to avoid photodegradation and thermal instability .
Q. Basic: What safety measures are essential when working with GdCl₃·6H₂O?
Use nitrile or neoprene gloves, goggles, and lab coats to prevent skin/eye contact. Employ fume hoods for synthesis steps to avoid inhalation of fine particulates. In case of exposure, rinse skin with water for 15 minutes; for eye contact, use emergency eyewash stations immediately. Dispose of waste via approved hazardous chemical protocols, avoiding direct environmental release .
Q. Advanced: How to design experiments for synthesizing Gd³⁺-doped upconverting nanoparticles?
Methodology :
- Use co-precipitation or thermal decomposition. For example, combine GdCl₃·6H₂O (99.99% purity) with YCl₃·6H₂O and Yb³⁺/Tm³⁺ chlorides in a 1-octadecene/oleic acid mixture under nitrogen .
- Control doping concentration (e.g., 5–20 mol% Gd³⁺) to optimize luminescence intensity and crystal phase (e.g., hexagonal vs. cubic Na(Y,Gd)F₄) .
- Purify nanoparticles via ethanol centrifugation and characterize using TEM (size), XRD (crystallinity), and photoluminescence spectroscopy (emission profiles) .
Q. Advanced: How to optimize Gd³⁺ doping levels in materials for magnetic or optical applications?
- Magnetic studies : Vary Gd³⁺ concentrations (0–100%) in host matrices (e.g., garnets or oxides) and measure magnetic susceptibility (χ) via SQUID magnetometry. Higher Gd³⁺ increases χ due to its 7 unpaired electrons .
- Optical studies : For upconversion, balance Gd³⁺ content to avoid cross-relaxation quenching. Use Judd-Ofelt theory to model radiative transitions and validate with lifetime decay measurements .
Q. Advanced: What techniques are used to analyze GdCl₃·6H₂O’s magnetic properties?
- DC magnetometry : Measure magnetization (M) vs. field (H) at 2–300 K to determine paramagnetic behavior.
- AC susceptibility : Detect spin-glass transitions or magnetic anisotropy.
- EPR spectroscopy : Identify Gd³⁺ electron spin states and local symmetry distortions .
Note: Correct for diamagnetic contributions from the hydrate using Pascal’s constants .
Q. Advanced: How to resolve contradictions in literature regarding GdCl₃·6H₂O’s efficacy in MRI contrast agents?
- Key variables : Purity (≥99.9%), hydration state, and colloidal stability in physiological buffers.
- Experimental controls :
- Address discrepancies : Reproduce studies under identical pH, temperature, and field strength (e.g., 1.5T vs. 3T MRI systems) .
Q. Methodological: How to ensure purity and characterize impurities in GdCl₃·6H₂O?
Analytical Workflow :
Inductively Coupled Plasma Mass Spectrometry (ICP-MS) : Quantify TREO (Total Rare Earth Oxides) and detect trace metals (e.g., Fe, Ca).
Thermogravimetric Analysis (TGA) : Confirm hydrate content (6H₂O) by measuring weight loss at 100–200°C.
X-ray Fluorescence (XRF) : Verify stoichiometry (Gd:Cl ≈ 1:3).
Typical Purity Specifications (3N Grade) :
Parameter | Value (wt%) |
---|---|
Gd₂O₃/TREO | ≥99.9% |
Fe₂O₃ | ≤0.001% |
SiO₂ | ≤0.002% |
CaO | ≤0.005% |
Source: |
Q. Methodological: What role does GdCl₃·6H₂O play in spectroscopic studies?
- Luminescence quenching : Use as a co-dopant in Eu³⁺/Tb³⁺ systems to study energy transfer via Förster resonance.
- EPR probes : Gd³⁺’s S=7/2 spin state enables high-sensitivity detection of local oxygen gradients in biological samples .
- FTIR analysis : Monitor ligand coordination shifts (e.g., OH stretching in hydrate at 3400 cm⁻¹) to assess hydration stability .
Properties
CAS No. |
13450-84-5 |
---|---|
Molecular Formula |
Cl3GdH2O |
Molecular Weight |
281.6 g/mol |
IUPAC Name |
trichlorogadolinium;hydrate |
InChI |
InChI=1S/3ClH.Gd.H2O/h3*1H;;1H2/q;;;+3;/p-3 |
InChI Key |
SXVCBXHAHBCOMV-UHFFFAOYSA-K |
SMILES |
O.O.O.O.O.O.[Cl-].[Cl-].[Cl-].[Gd+3] |
Canonical SMILES |
O.Cl[Gd](Cl)Cl |
Pictograms |
Irritant |
Origin of Product |
United States |
Retrosynthesis Analysis
AI-Powered Synthesis Planning: Our tool employs the Template_relevance Pistachio, Template_relevance Bkms_metabolic, Template_relevance Pistachio_ringbreaker, Template_relevance Reaxys, Template_relevance Reaxys_biocatalysis model, leveraging a vast database of chemical reactions to predict feasible synthetic routes.
One-Step Synthesis Focus: Specifically designed for one-step synthesis, it provides concise and direct routes for your target compounds, streamlining the synthesis process.
Accurate Predictions: Utilizing the extensive PISTACHIO, BKMS_METABOLIC, PISTACHIO_RINGBREAKER, REAXYS, REAXYS_BIOCATALYSIS database, our tool offers high-accuracy predictions, reflecting the latest in chemical research and data.
Strategy Settings
Precursor scoring | Relevance Heuristic |
---|---|
Min. plausibility | 0.01 |
Model | Template_relevance |
Template Set | Pistachio/Bkms_metabolic/Pistachio_ringbreaker/Reaxys/Reaxys_biocatalysis |
Top-N result to add to graph | 6 |
Feasible Synthetic Routes
Disclaimer and Information on In-Vitro Research Products
Please be aware that all articles and product information presented on BenchChem are intended solely for informational purposes. The products available for purchase on BenchChem are specifically designed for in-vitro studies, which are conducted outside of living organisms. In-vitro studies, derived from the Latin term "in glass," involve experiments performed in controlled laboratory settings using cells or tissues. It is important to note that these products are not categorized as medicines or drugs, and they have not received approval from the FDA for the prevention, treatment, or cure of any medical condition, ailment, or disease. We must emphasize that any form of bodily introduction of these products into humans or animals is strictly prohibited by law. It is essential to adhere to these guidelines to ensure compliance with legal and ethical standards in research and experimentation.