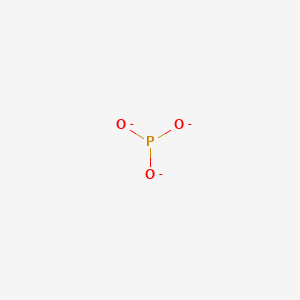
Phosphite
Overview
Description
Phosphite is a chemical compound containing the this compound ion, which is represented as PO₃³⁻. It is commonly found in various salts and esters. Phosphites are known for their role in organic and inorganic chemistry, particularly in the synthesis of other phosphorus-containing compounds. They are often used as reducing agents and stabilizers in various chemical processes .
Preparation Methods
Synthetic Routes and Reaction Conditions: Phosphites can be synthesized through several methods, including the hydrolysis of phosphorus trichloride (PCl₃). The reaction involves the addition of water to phosphorus trichloride, resulting in the formation of phosphorous acid (H₃PO₃) and hydrochloric acid (HCl): [ \text{PCl}_3 + 3\text{H}_2\text{O} \rightarrow \text{H}_3\text{PO}_3 + 3\text{HCl} ] The resulting solution is then heated to remove hydrochloric acid and evaporate the remaining water, yielding colorless crystalline phosphorous acid .
Industrial Production Methods: In industrial settings, phosphites are often produced through the reduction of phosphates or the reaction of phosphorous acid with bases. These methods are scalable and efficient, allowing for the mass production of phosphite compounds .
Chemical Reactions Analysis
Types of Reactions: Phosphites undergo various chemical reactions, including:
Oxidation: Phosphites can be oxidized to phosphates using oxidizing agents such as hydrogen peroxide (H₂O₂).
Reduction: Phosphites act as reducing agents in reactions with other compounds.
Substitution: Phosphites can participate in substitution reactions, where the phosphite ion replaces another group in a compound.
Common Reagents and Conditions:
Oxidation: Hydrogen peroxide (H₂O₂) is commonly used as an oxidizing agent.
Reduction: Phosphites themselves act as reducing agents.
Substitution: Various organic and inorganic reagents can be used, depending on the specific reaction.
Major Products:
Oxidation: Phosphates (PO₄³⁻)
Reduction: Reduced forms of the reacting compounds
Substitution: Substituted this compound derivatives
Scientific Research Applications
Phosphites have a wide range of applications in scientific research:
Chemistry: Used as reducing agents and stabilizers in various chemical reactions.
Biology: Involved in the synthesis of nucleotides and other biologically relevant molecules.
Medicine: Investigated for their potential use in drug development and as antioxidants.
Industry: Employed as stabilizers in polymer production and as additives in fertilizers to enhance nutrient uptake in plants
Mechanism of Action
Phosphites are often compared with other phosphorus-containing compounds such as phosphates and phosphonates. While phosphates (PO₄³⁻) are fully oxidized and stable, phosphites (PO₃³⁻) are less oxidized and more reactive. Phosphonates (R-PO₃²⁻) contain a carbon-phosphorus bond, making them distinct in their chemical behavior and applications .
Comparison with Similar Compounds
- Phosphates (PO₄³⁻)
- Phosphonates (R-PO₃²⁻)
- Phosphines (PH₃)
- Phosphonium Salts (R₄P⁺)
Phosphites are unique due to their intermediate oxidation state and their ability to act as both reducing agents and stabilizers, which sets them apart from other phosphorus compounds .
Properties
IUPAC Name |
phosphite | |
---|---|---|
Source | PubChem | |
URL | https://pubchem.ncbi.nlm.nih.gov | |
Description | Data deposited in or computed by PubChem | |
InChI |
InChI=1S/O3P/c1-4(2)3/q-3 | |
Source | PubChem | |
URL | https://pubchem.ncbi.nlm.nih.gov | |
Description | Data deposited in or computed by PubChem | |
InChI Key |
AQSJGOWTSHOLKH-UHFFFAOYSA-N | |
Source | PubChem | |
URL | https://pubchem.ncbi.nlm.nih.gov | |
Description | Data deposited in or computed by PubChem | |
Canonical SMILES |
[O-]P([O-])[O-] | |
Source | PubChem | |
URL | https://pubchem.ncbi.nlm.nih.gov | |
Description | Data deposited in or computed by PubChem | |
Molecular Formula |
O3P-3 | |
Source | PubChem | |
URL | https://pubchem.ncbi.nlm.nih.gov | |
Description | Data deposited in or computed by PubChem | |
DSSTOX Substance ID |
DTXSID80164154 | |
Record name | Phosphite | |
Source | EPA DSSTox | |
URL | https://comptox.epa.gov/dashboard/DTXSID80164154 | |
Description | DSSTox provides a high quality public chemistry resource for supporting improved predictive toxicology. | |
Molecular Weight |
78.972 g/mol | |
Source | PubChem | |
URL | https://pubchem.ncbi.nlm.nih.gov | |
Description | Data deposited in or computed by PubChem | |
Physical Description |
Other Solid, Black granular powder; [National Institute of Standards and Technology MSDS] | |
Record name | Phosphate rock and Phosphorite, calcined | |
Source | EPA Chemicals under the TSCA | |
URL | https://www.epa.gov/chemicals-under-tsca | |
Description | EPA Chemicals under the Toxic Substances Control Act (TSCA) collection contains information on chemicals and their regulations under TSCA, including non-confidential content from the TSCA Chemical Substance Inventory and Chemical Data Reporting. | |
Record name | Phosphorite | |
Source | Haz-Map, Information on Hazardous Chemicals and Occupational Diseases | |
URL | https://haz-map.com/Agents/21482 | |
Description | Haz-Map® is an occupational health database designed for health and safety professionals and for consumers seeking information about the adverse effects of workplace exposures to chemical and biological agents. | |
Explanation | Copyright (c) 2022 Haz-Map(R). All rights reserved. Unless otherwise indicated, all materials from Haz-Map are copyrighted by Haz-Map(R). No part of these materials, either text or image may be used for any purpose other than for personal use. Therefore, reproduction, modification, storage in a retrieval system or retransmission, in any form or by any means, electronic, mechanical or otherwise, for reasons other than personal use, is strictly prohibited without prior written permission. | |
CAS No. |
14901-63-4, 65996-94-3 | |
Record name | Phosphite | |
Source | CAS Common Chemistry | |
URL | https://commonchemistry.cas.org/detail?cas_rn=14901-63-4 | |
Description | CAS Common Chemistry is an open community resource for accessing chemical information. Nearly 500,000 chemical substances from CAS REGISTRY cover areas of community interest, including common and frequently regulated chemicals, and those relevant to high school and undergraduate chemistry classes. This chemical information, curated by our expert scientists, is provided in alignment with our mission as a division of the American Chemical Society. | |
Explanation | The data from CAS Common Chemistry is provided under a CC-BY-NC 4.0 license, unless otherwise stated. | |
Record name | Phosphite | |
Source | ChemIDplus | |
URL | https://pubchem.ncbi.nlm.nih.gov/substance/?source=chemidplus&sourceid=0014901634 | |
Description | ChemIDplus is a free, web search system that provides access to the structure and nomenclature authority files used for the identification of chemical substances cited in National Library of Medicine (NLM) databases, including the TOXNET system. | |
Record name | Phosphate rock and Phosphorite, calcined | |
Source | EPA Chemicals under the TSCA | |
URL | https://www.epa.gov/chemicals-under-tsca | |
Description | EPA Chemicals under the Toxic Substances Control Act (TSCA) collection contains information on chemicals and their regulations under TSCA, including non-confidential content from the TSCA Chemical Substance Inventory and Chemical Data Reporting. | |
Record name | Phosphite | |
Source | EPA DSSTox | |
URL | https://comptox.epa.gov/dashboard/DTXSID80164154 | |
Description | DSSTox provides a high quality public chemistry resource for supporting improved predictive toxicology. | |
Record name | Phosphate rock and Phosphorite, calcined | |
Source | European Chemicals Agency (ECHA) | |
URL | https://echa.europa.eu/substance-information/-/substanceinfo/100.060.008 | |
Description | The European Chemicals Agency (ECHA) is an agency of the European Union which is the driving force among regulatory authorities in implementing the EU's groundbreaking chemicals legislation for the benefit of human health and the environment as well as for innovation and competitiveness. | |
Explanation | Use of the information, documents and data from the ECHA website is subject to the terms and conditions of this Legal Notice, and subject to other binding limitations provided for under applicable law, the information, documents and data made available on the ECHA website may be reproduced, distributed and/or used, totally or in part, for non-commercial purposes provided that ECHA is acknowledged as the source: "Source: European Chemicals Agency, http://echa.europa.eu/". Such acknowledgement must be included in each copy of the material. ECHA permits and encourages organisations and individuals to create links to the ECHA website under the following cumulative conditions: Links can only be made to webpages that provide a link to the Legal Notice page. | |
Synthesis routes and methods I
Procedure details
Synthesis routes and methods II
Procedure details
Disclaimer and Information on In-Vitro Research Products
Please be aware that all articles and product information presented on BenchChem are intended solely for informational purposes. The products available for purchase on BenchChem are specifically designed for in-vitro studies, which are conducted outside of living organisms. In-vitro studies, derived from the Latin term "in glass," involve experiments performed in controlled laboratory settings using cells or tissues. It is important to note that these products are not categorized as medicines or drugs, and they have not received approval from the FDA for the prevention, treatment, or cure of any medical condition, ailment, or disease. We must emphasize that any form of bodily introduction of these products into humans or animals is strictly prohibited by law. It is essential to adhere to these guidelines to ensure compliance with legal and ethical standards in research and experimentation.