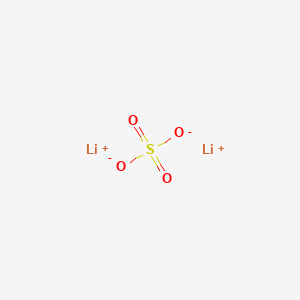
Lithium sulfate
Overview
Description
Lithium sulfate is a white inorganic salt with the formula Li2SO4 . It is the lithium salt of sulfuric acid . It is a colorless monoclinic or hexagonal crystalline solid . Although lithium sulfate solution is prepared in a large quantity as an intermediate in the spodumene-sulfuric acid process, the pure compound has only limited industrial use .
Synthesis Analysis
Lithium sulfate can be synthesized via spontaneous metathesis reactions between lithium salts (halides and nitrate) and sodium sulfide . This innovative method is economical, scalable, and green . Another method involves the conversion of β spodumene to lithium sulfate using concentrated sulfuric acid .Molecular Structure Analysis
Lithium sulfate has a primitive monoclinic crystal structure . The edge lengths of the unit cell are a = 8.239 Å, b = 4.954 Å, c = 8.474 Å, and β = 107.98° .Chemical Reactions Analysis
The sulfur reduction reaction in a lithium-sulfur battery involves 16 electrons to convert an eight-atom sulfur ring molecule into lithium sulfide in a catalytic reaction network with numerous interwoven branches and different intermediate products called lithium polysulfides and many other byproducts . The reactions can be represented as: 2LiOH + H2SO4 → Li2SO4 + H2O and Li2CO3 + H2SO4 → Li2SO4 + CO2 + H2O .Physical And Chemical Properties Analysis
Lithium sulfate has a molar mass of 109.94 g/mol . It appears as a white crystalline solid and is hygroscopic . It has a density of 2.221 g/cm3 (anhydrous) and 2.06 g/cm3 (monohydrate) . It has a melting point of 859 °C and a boiling point of 1,377 °C . It is soluble in water but its solubility decreases with increasing temperature . It is insoluble in absolute ethanol, acetone, and pyridine .Scientific Research Applications
Waste Treatment and Resource Recovery : Gao et al. (2021) developed bipolar membrane electrodialysis (BMED) technology for treating waste sodium sulfate containing lithium carbonate. This process converts sodium sulfate into sulfuric acid and sodium hydroxide, which can be reused in upstream processes. The research indicates potential economic and environmental benefits of this technology (Gao et al., 2021).
Battery Technology : Zhang et al. (2022) presented a novel method for synthesizing lithium sulfide (Li2S) from lithium sulfate, which is crucial for lithium-sulfur batteries and all-solid-state batteries. This method offers a green, cost-effective alternative to conventional methods, with potential applications in energy storage and conversion (Zhang et al., 2022).
Bioleaching in Battery Recycling : Heydarian et al. (2018) explored bioleaching of spent lithium-ion batteries using a mixture of acidophilic bacteria. This process demonstrated high efficiency in metal recovery, including lithium, and presents an environmentally friendly approach to recycling lithium-ion batteries (Heydarian et al., 2018).
Medicinal Applications : Miyamoto et al. (2000) identified lithium as an in vivo target in fission yeast. Their study suggested that lithium-sensitive phosphomonoesterase might be a likely in vivo target of lithium action across species, offering insights into lithium therapy for bipolar affective disorder (Miyamoto et al., 2000).
Construction Materials : Demir and Sevim (2017) investigated the influence of lithium sulfate on the properties of Portland cement paste. The study found that lithium sulfate accelerates cement hydration, enhances strength, and shortens setting time (Demir & Sevim, 2017).
Lithium Hydroxide Production : Chen et al. (2021) explored the conversion of lithium sulfate to lithium hydroxide using electrodialysis with bipolar membranes. This process could replace chemical precipitation techniques in producing lithium hydroxide, commonly used in lithium-ion batteries (Chen et al., 2021).
Lithium Enrichment in Agriculture : Silva et al. (2019) researched the biofortification of lettuce with lithium using lithium sulfate. They found that lower concentrations of lithium sulfate could enhance plant growth and increase lithium accumulation in plants (Silva et al., 2019).
Mechanism of Action
Safety and Hazards
properties
IUPAC Name |
dilithium;sulfate | |
---|---|---|
Source | PubChem | |
URL | https://pubchem.ncbi.nlm.nih.gov | |
Description | Data deposited in or computed by PubChem | |
InChI |
InChI=1S/2Li.H2O4S/c;;1-5(2,3)4/h;;(H2,1,2,3,4)/q2*+1;/p-2 | |
Source | PubChem | |
URL | https://pubchem.ncbi.nlm.nih.gov | |
Description | Data deposited in or computed by PubChem | |
InChI Key |
INHCSSUBVCNVSK-UHFFFAOYSA-L | |
Source | PubChem | |
URL | https://pubchem.ncbi.nlm.nih.gov | |
Description | Data deposited in or computed by PubChem | |
Canonical SMILES |
[Li+].[Li+].[O-]S(=O)(=O)[O-] | |
Source | PubChem | |
URL | https://pubchem.ncbi.nlm.nih.gov | |
Description | Data deposited in or computed by PubChem | |
Molecular Formula |
Li2SO4, Li2O4S | |
Record name | lithium sulfate | |
Source | Wikipedia | |
URL | https://en.wikipedia.org/wiki/Lithium_sulfate | |
Description | Chemical information link to Wikipedia. | |
Source | PubChem | |
URL | https://pubchem.ncbi.nlm.nih.gov | |
Description | Data deposited in or computed by PubChem | |
DSSTOX Substance ID |
DTXSID3049201 | |
Record name | Lithium sulfate | |
Source | EPA DSSTox | |
URL | https://comptox.epa.gov/dashboard/DTXSID3049201 | |
Description | DSSTox provides a high quality public chemistry resource for supporting improved predictive toxicology. | |
Molecular Weight |
110.0 g/mol | |
Source | PubChem | |
URL | https://pubchem.ncbi.nlm.nih.gov | |
Description | Data deposited in or computed by PubChem | |
Product Name |
Lithium sulfate | |
CAS RN |
10377-48-7 | |
Record name | Lithium sulfate | |
Source | ChemIDplus | |
URL | https://pubchem.ncbi.nlm.nih.gov/substance/?source=chemidplus&sourceid=0010377487 | |
Description | ChemIDplus is a free, web search system that provides access to the structure and nomenclature authority files used for the identification of chemical substances cited in National Library of Medicine (NLM) databases, including the TOXNET system. | |
Record name | Sulfuric acid, lithium salt (1:2) | |
Source | EPA Chemicals under the TSCA | |
URL | https://www.epa.gov/chemicals-under-tsca | |
Description | EPA Chemicals under the Toxic Substances Control Act (TSCA) collection contains information on chemicals and their regulations under TSCA, including non-confidential content from the TSCA Chemical Substance Inventory and Chemical Data Reporting. | |
Record name | Lithium sulfate | |
Source | EPA DSSTox | |
URL | https://comptox.epa.gov/dashboard/DTXSID3049201 | |
Description | DSSTox provides a high quality public chemistry resource for supporting improved predictive toxicology. | |
Record name | Lithium sulphate | |
Source | European Chemicals Agency (ECHA) | |
URL | https://echa.europa.eu/substance-information/-/substanceinfo/100.030.734 | |
Description | The European Chemicals Agency (ECHA) is an agency of the European Union which is the driving force among regulatory authorities in implementing the EU's groundbreaking chemicals legislation for the benefit of human health and the environment as well as for innovation and competitiveness. | |
Explanation | Use of the information, documents and data from the ECHA website is subject to the terms and conditions of this Legal Notice, and subject to other binding limitations provided for under applicable law, the information, documents and data made available on the ECHA website may be reproduced, distributed and/or used, totally or in part, for non-commercial purposes provided that ECHA is acknowledged as the source: "Source: European Chemicals Agency, http://echa.europa.eu/". Such acknowledgement must be included in each copy of the material. ECHA permits and encourages organisations and individuals to create links to the ECHA website under the following cumulative conditions: Links can only be made to webpages that provide a link to the Legal Notice page. | |
Record name | LITHIUM SULFATE | |
Source | FDA Global Substance Registration System (GSRS) | |
URL | https://gsrs.ncats.nih.gov/ginas/app/beta/substances/919XA137JK | |
Description | The FDA Global Substance Registration System (GSRS) enables the efficient and accurate exchange of information on what substances are in regulated products. Instead of relying on names, which vary across regulatory domains, countries, and regions, the GSRS knowledge base makes it possible for substances to be defined by standardized, scientific descriptions. | |
Explanation | Unless otherwise noted, the contents of the FDA website (www.fda.gov), both text and graphics, are not copyrighted. They are in the public domain and may be republished, reprinted and otherwise used freely by anyone without the need to obtain permission from FDA. Credit to the U.S. Food and Drug Administration as the source is appreciated but not required. | |
Synthesis routes and methods
Procedure details
Retrosynthesis Analysis
AI-Powered Synthesis Planning: Our tool employs the Template_relevance Pistachio, Template_relevance Bkms_metabolic, Template_relevance Pistachio_ringbreaker, Template_relevance Reaxys, Template_relevance Reaxys_biocatalysis model, leveraging a vast database of chemical reactions to predict feasible synthetic routes.
One-Step Synthesis Focus: Specifically designed for one-step synthesis, it provides concise and direct routes for your target compounds, streamlining the synthesis process.
Accurate Predictions: Utilizing the extensive PISTACHIO, BKMS_METABOLIC, PISTACHIO_RINGBREAKER, REAXYS, REAXYS_BIOCATALYSIS database, our tool offers high-accuracy predictions, reflecting the latest in chemical research and data.
Strategy Settings
Precursor scoring | Relevance Heuristic |
---|---|
Min. plausibility | 0.01 |
Model | Template_relevance |
Template Set | Pistachio/Bkms_metabolic/Pistachio_ringbreaker/Reaxys/Reaxys_biocatalysis |
Top-N result to add to graph | 6 |
Feasible Synthetic Routes
Disclaimer and Information on In-Vitro Research Products
Please be aware that all articles and product information presented on BenchChem are intended solely for informational purposes. The products available for purchase on BenchChem are specifically designed for in-vitro studies, which are conducted outside of living organisms. In-vitro studies, derived from the Latin term "in glass," involve experiments performed in controlled laboratory settings using cells or tissues. It is important to note that these products are not categorized as medicines or drugs, and they have not received approval from the FDA for the prevention, treatment, or cure of any medical condition, ailment, or disease. We must emphasize that any form of bodily introduction of these products into humans or animals is strictly prohibited by law. It is essential to adhere to these guidelines to ensure compliance with legal and ethical standards in research and experimentation.