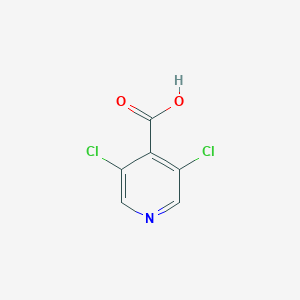
3,5-Dichloroisonicotinic acid
Overview
Description
Synthesis Analysis
The synthesis of 3,5-Dichloroisonicotinic acid or its related compounds typically involves reactions such as oxidation, chlorination, and hydrolysis. For instance, Shi Wei-bing (2009) describes the synthesis of 2-Chloronicotinic acid, a related compound, from 3-cyanopyridine through these reactions, achieving a total yield of 75.1% (Shi Wei-bing, 2009). Similarly, Wei Xiao-lei (2010) achieved a total yield of 77.2% and purity of 99.5% under optimized reaction conditions for the same compound (Wei Xiao-lei, 2010).
Molecular Structure Analysis
The molecular structure of compounds like 3,5-Dichloroisonicotinic acid is often studied using techniques like infrared (IR) and Raman spectroscopy. For example, a study by M. Karabacak and M. Kurt (2008) on 6-chloronicotinic acid used Fourier transform infrared and Raman spectra to analyze the molecular structure, identifying various conformers and their stability (Karabacak & Kurt, 2008).
Chemical Reactions and Properties
The reactivity of 3,5-Dichloroisonicotinic acid can be inferred from studies on similar compounds. For example, Qianli Li et al. (2015) synthesized organostannoxanes derived from 2-chloroisonicotinic acid, revealing insights into its reactivity and the formation of complex structures (Li et al., 2015).
Physical Properties Analysis
The physical properties of 3,5-Dichloroisonicotinic acid are closely related to its molecular structure. S. Long et al. (2015) explored the polymorphism and phase transitions of 3-chloroisonicotinic acid, highlighting its diverse physical forms and stability (Long et al., 2015).
Chemical Properties Analysis
The chemical properties of this compound can be partly understood through its synthesis and molecular structure. Studies like those by M. Karabacak et al. (2008) provide insight into the chemical behavior and properties of chloronicotinic acids (Karabacak et al., 2008).
Scientific Research Applications
Radiolabeling and Bioconjugate Synthesis : Analogues of 3,5-Dichloroisonicotinic acid, such as HYNIC (Hydrazinonicotinic acid), have been explored for their ability to chelate technetium in radiolabeling applications. These compounds are used in synthesizing bioconjugates for radiolabeling with Tc-99m, useful in nuclear medicine for imaging and diagnostic purposes (Meszaros et al., 2011).
Agricultural Applications : 3,5-Dichloroisonicotinic acid has been used to treat soybeans, leading to reduced severity of white mold disease caused by Sclerotinia sclerotiorum in field and greenhouse studies. This suggests its potential role as a protective agent against plant diseases (Dann et al., 1998).
Synthesis of Antibiotics and Food Additives : The compound has been synthesized as a constituent of the orthosomycin antibiotics avilamycin, curamycin, everninomycin, and related derivatives. It is also known as a food additive (Dornhagen & Scharf, 1985).
Material Science : In the synthesis of heterometallic uranyl-silver coordination polymers, 3,5-Dichloroisonicotinic acid is used to promote structure variance. It plays a significant role in the formation of three-dimensional frameworks in these polymers (Mei et al., 2018).
Pesticide and Medicine Precursors : It serves as a key precursor in the synthesis of pesticides and medicines, particularly in transformations involving Rhodococcus erythropolis, which converts related compounds into useful intermediates (Jin et al., 2011).
Postharvest Treatment in Citrus Fruits : Treating citrus fruits with 3,5-Dichloroisonicotinic acid has been shown to reduce postharvest diseases like blue and green molds and anthracnose decay. It also enhances defense-related enzymes in the fruits (Jing et al., 2020).
Elicitor for Plant Secondary Metabolites : As an elicitor, 3,5-Dichloroisonicotinic acid and its derivatives have been used to induce the biosynthesis of secondary metabolites in plants, which can be important for pharmaceutical applications (Qian et al., 2006).
Biochemical Research : In biochemical assays, 3,5-Dichloroisonicotinic acid has been used in chromogenic systems for measuring substances like hydrogen peroxide, indicating its utility in laboratory analyses (Fossati & Prencipe, 2010).
Safety And Hazards
Future Directions
One of the potential future directions for 3,5-Dichloroisonicotinic Acid and its derivatives is in the field of plant disease resistance. Ester derivatives of nicotinic, isonicotinic, and 2,6-dichloroisonicotinic acids have been studied for their potential to induce plants’ natural immune system .
Relevant Papers One relevant paper is “Halogen Bonded Three-Dimensional Uranyl–Organic Compounds with Unprecedented Halogen–Halogen Interactions and Structure Diversity upon Variation of Halogen Substitution” published in Crystal Growth & Design in 2015 . Another paper titled “Simple modifications of nicotinic, isonicotinic, and 2,6-dichloroisonicotinic acids toward new weapons against plant diseases” discusses the potential use of 3,5-Dichloroisonicotinic Acid in plant disease resistance .
properties
IUPAC Name |
3,5-dichloropyridine-4-carboxylic acid | |
---|---|---|
Source | PubChem | |
URL | https://pubchem.ncbi.nlm.nih.gov | |
Description | Data deposited in or computed by PubChem | |
InChI |
InChI=1S/C6H3Cl2NO2/c7-3-1-9-2-4(8)5(3)6(10)11/h1-2H,(H,10,11) | |
Source | PubChem | |
URL | https://pubchem.ncbi.nlm.nih.gov | |
Description | Data deposited in or computed by PubChem | |
InChI Key |
BUQPTOSHKHYHHB-UHFFFAOYSA-N | |
Source | PubChem | |
URL | https://pubchem.ncbi.nlm.nih.gov | |
Description | Data deposited in or computed by PubChem | |
Canonical SMILES |
C1=C(C(=C(C=N1)Cl)C(=O)O)Cl | |
Source | PubChem | |
URL | https://pubchem.ncbi.nlm.nih.gov | |
Description | Data deposited in or computed by PubChem | |
Molecular Formula |
C6H3Cl2NO2 | |
Source | PubChem | |
URL | https://pubchem.ncbi.nlm.nih.gov | |
Description | Data deposited in or computed by PubChem | |
DSSTOX Substance ID |
DTXSID20345602 | |
Record name | 3,5-Dichloroisonicotinic acid | |
Source | EPA DSSTox | |
URL | https://comptox.epa.gov/dashboard/DTXSID20345602 | |
Description | DSSTox provides a high quality public chemistry resource for supporting improved predictive toxicology. | |
Molecular Weight |
192.00 g/mol | |
Source | PubChem | |
URL | https://pubchem.ncbi.nlm.nih.gov | |
Description | Data deposited in or computed by PubChem | |
Product Name |
3,5-Dichloroisonicotinic acid | |
CAS RN |
13958-93-5 | |
Record name | 3,5-Dichloroisonicotinic acid | |
Source | EPA DSSTox | |
URL | https://comptox.epa.gov/dashboard/DTXSID20345602 | |
Description | DSSTox provides a high quality public chemistry resource for supporting improved predictive toxicology. | |
Record name | 3,5-Dichloroisonicotinic acid | |
Source | European Chemicals Agency (ECHA) | |
URL | https://echa.europa.eu/information-on-chemicals | |
Description | The European Chemicals Agency (ECHA) is an agency of the European Union which is the driving force among regulatory authorities in implementing the EU's groundbreaking chemicals legislation for the benefit of human health and the environment as well as for innovation and competitiveness. | |
Explanation | Use of the information, documents and data from the ECHA website is subject to the terms and conditions of this Legal Notice, and subject to other binding limitations provided for under applicable law, the information, documents and data made available on the ECHA website may be reproduced, distributed and/or used, totally or in part, for non-commercial purposes provided that ECHA is acknowledged as the source: "Source: European Chemicals Agency, http://echa.europa.eu/". Such acknowledgement must be included in each copy of the material. ECHA permits and encourages organisations and individuals to create links to the ECHA website under the following cumulative conditions: Links can only be made to webpages that provide a link to the Legal Notice page. | |
Synthesis routes and methods I
Procedure details
Synthesis routes and methods II
Procedure details
Retrosynthesis Analysis
AI-Powered Synthesis Planning: Our tool employs the Template_relevance Pistachio, Template_relevance Bkms_metabolic, Template_relevance Pistachio_ringbreaker, Template_relevance Reaxys, Template_relevance Reaxys_biocatalysis model, leveraging a vast database of chemical reactions to predict feasible synthetic routes.
One-Step Synthesis Focus: Specifically designed for one-step synthesis, it provides concise and direct routes for your target compounds, streamlining the synthesis process.
Accurate Predictions: Utilizing the extensive PISTACHIO, BKMS_METABOLIC, PISTACHIO_RINGBREAKER, REAXYS, REAXYS_BIOCATALYSIS database, our tool offers high-accuracy predictions, reflecting the latest in chemical research and data.
Strategy Settings
Precursor scoring | Relevance Heuristic |
---|---|
Min. plausibility | 0.01 |
Model | Template_relevance |
Template Set | Pistachio/Bkms_metabolic/Pistachio_ringbreaker/Reaxys/Reaxys_biocatalysis |
Top-N result to add to graph | 6 |
Feasible Synthetic Routes
Q & A
Q1: How does the chlorination of isonicotinic acid impact the formation of uranyl-silver coordination polymers?
A1: The research by [] demonstrates that stepwise ortho-chlorination of the carboxyl group in isonicotinic acid significantly influences the resulting structure of uranyl-silver coordination polymers. The introduction of chlorine atoms, particularly in the 3 and 5 positions of the aromatic ring, introduces steric hindrance. This steric effect alters the typical coordination behavior of the carboxyl group. Instead of primarily interacting with uranyl ions as seen with unsubstituted isonicotinic acid, the carboxyl group in 3,5-dichloroisonicotinic acid participates in coordination with silver ions. This change in coordination preference leads to the formation of distinct 3D heterometallic frameworks with highly coordinated silver nodes. These structures differ significantly from those formed with unchlorinated or less chlorinated isonicotinic acid derivatives, highlighting the importance of chlorine substitution in directing the self-assembly process. []
Q2: What are the structural characteristics of the uranyl-silver framework formed with 3,5-dichloroisonicotinic acid?
A2: The research describes the formation of a unique 3D heterometallic framework, (UO2)Ag4(3,5-DCPCA)6(3,5-DCPy)2, using 3,5-dichloroisonicotinic acid (3,5-DCPCA) []. In this structure, 3,5-DCPCA acts as a bridging ligand, coordinating to both uranyl and silver ions. Interestingly, the framework also incorporates in situ generated 3,5-dichloropyridine (3,5-DCPy), further demonstrating the influence of chlorine substitution on the system's chemistry. The presence of multiple argentophilic interactions contributes to the overall stability of the 3D framework. This structure contrasts with the frameworks formed with 3-chloroisonicotinic acid, which while also three-dimensional, exhibit different silver coordination environments and overall connectivity. []
Disclaimer and Information on In-Vitro Research Products
Please be aware that all articles and product information presented on BenchChem are intended solely for informational purposes. The products available for purchase on BenchChem are specifically designed for in-vitro studies, which are conducted outside of living organisms. In-vitro studies, derived from the Latin term "in glass," involve experiments performed in controlled laboratory settings using cells or tissues. It is important to note that these products are not categorized as medicines or drugs, and they have not received approval from the FDA for the prevention, treatment, or cure of any medical condition, ailment, or disease. We must emphasize that any form of bodily introduction of these products into humans or animals is strictly prohibited by law. It is essential to adhere to these guidelines to ensure compliance with legal and ethical standards in research and experimentation.