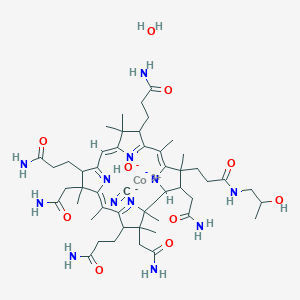
Vitamin B12 factor B
Overview
Description
Vitamin B12, also known as cobalamin, is a water-soluble vitamin that plays a crucial role in the metabolism of every cell in the human body. It is involved in DNA synthesis, fatty acid and amino acid metabolism, and red blood cell formation. Vitamin B12 is unique among vitamins as it contains a metal ion, cobalt, at its core. This vitamin is essential for the proper functioning of the brain and nervous system, as well as for the formation of blood .
Preparation Methods
Synthetic Routes and Reaction Conditions: The chemical synthesis of Vitamin B12 is highly complex due to its intricate structure. The synthesis involves over 30 steps, making it one of the most challenging compounds to produce synthetically.
Industrial Production Methods: Industrial production of Vitamin B12 is primarily achieved through microbial fermentation. The most commonly used microorganisms for this purpose are strains of Propionibacterium freudenreichii and Pseudomonas denitrificans. These bacteria are cultivated in large fermentation tanks under controlled conditions, where they produce Vitamin B12 as a byproduct of their metabolic processes. The vitamin is then extracted and purified for use in supplements and fortified foods .
Chemical Reactions Analysis
Types of Reactions: Vitamin B12 undergoes several types of chemical reactions, including:
Oxidation and Reduction: The cobalt ion in Vitamin B12 can exist in multiple oxidation states, allowing it to participate in redox reactions.
Common Reagents and Conditions:
Oxidation: Hydrogen peroxide or other oxidizing agents can be used to oxidize Vitamin B12.
Reduction: Reducing agents like sodium borohydride can reduce the cobalt ion in Vitamin B12.
Major Products Formed:
Methylcobalamin: Formed by the substitution of the upper axial ligand with a methyl group.
Adenosylcobalamin: Formed by the substitution of the upper axial ligand with an adenosyl group.
Scientific Research Applications
Vitamin B12 has a wide range of applications in scientific research:
Biology: Essential for studying cellular metabolism, DNA synthesis, and the functioning of the nervous system.
Medicine: Used in the treatment of Vitamin B12 deficiency, which can lead to pernicious anemia and neurological disorders.
Industry: Used in the fortification of foods and in the production of dietary supplements.
Mechanism of Action
Vitamin B12 acts as a coenzyme for several important enzymes in the body:
Methionine Synthase: Catalyzes the conversion of homocysteine to methionine, which is essential for DNA methylation and synthesis.
Methylmalonyl-CoA Mutase: Converts methylmalonyl-CoA to succinyl-CoA, a critical step in the metabolism of certain amino acids and fatty acids.
The molecular targets of Vitamin B12 include enzymes involved in DNA synthesis and repair, as well as those involved in the metabolism of fatty acids and amino acids. The pathways influenced by Vitamin B12 are crucial for maintaining cellular health and preventing genetic instability .
Comparison with Similar Compounds
Hydroxocobalamin: A form of Vitamin B12 used in the treatment of cyanide poisoning and Vitamin B12 deficiency.
Cyanocobalamin: The most stable and commonly used form of Vitamin B12 in supplements.
Methylcobalamin: A biologically active form of Vitamin B12 that is readily utilized by the body.
Adenosylcobalamin: Another biologically active form of Vitamin B12 involved in energy production.
Uniqueness: Vitamin B12 is unique due to its complex structure, which includes a corrin ring and a central cobalt ion. This structure allows it to participate in a wide range of biochemical reactions that are essential for human health. Unlike other vitamins, Vitamin B12 is not synthesized by plants or animals but is produced by certain bacteria and archaea .
Biological Activity
Vitamin B12, also known as cobalamin, is a water-soluble vitamin essential for various biological processes in the human body. Among its multiple forms, methylcobalamin and 5-deoxyadenosylcobalamin are the active coenzyme forms that play critical roles in metabolism, particularly in DNA synthesis, red blood cell formation, and neurological function. This article delves into the biological activity of Vitamin B12, specifically focusing on its mechanisms of action, clinical significance, and related case studies.
Vitamin B12 functions primarily as a cofactor for two key enzymes:
- Methionine Synthase : This enzyme catalyzes the conversion of homocysteine to methionine, an essential amino acid crucial for protein synthesis and methylation reactions. Methionine is also a precursor for S-adenosylmethionine (SAM), a universal methyl donor involved in the methylation of DNA, RNA, proteins, and lipids .
- L-Methylmalonyl-CoA Mutase : This enzyme facilitates the conversion of L-methylmalonyl-CoA to succinyl-CoA in the metabolism of certain fatty acids and amino acids. This reaction is vital for energy production and metabolic balance .
Absorption and Transport
The absorption of Vitamin B12 is a complex process involving several steps:
- Release from Food : Vitamin B12 is bound to proteins in food and must be released by gastric acid and proteases in the stomach.
- Binding to Transport Proteins : Once released, it binds to haptocorrin in saliva and later to intrinsic factor (IF) in the intestine. The Vitamin B12-IF complex is absorbed in the distal ileum through receptor-mediated endocytosis .
- Transport in Plasma : In circulation, Vitamin B12 is primarily bound to transcobalamin II (TC), which delivers it to tissues. Only about 20% of circulating Vitamin B12 is bound to TC; the rest is associated with haptocorrin .
Clinical Significance
Vitamin B12 deficiency can lead to significant health issues, including:
- Anemia : Macrocytic anemia occurs due to impaired red blood cell formation.
- Neurological Disorders : Deficiency can result in neuropathy, cognitive decline, and other neurological symptoms due to its role in myelin synthesis .
Table 1: Clinical Manifestations of Vitamin B12 Deficiency
Symptom/Condition | Description |
---|---|
Macrocytic Anemia | Enlarged red blood cells due to impaired division |
Peripheral Neuropathy | Nerve damage leading to weakness and numbness |
Cognitive Impairment | Memory loss and cognitive decline |
Glossitis | Inflammation of the tongue |
Case Studies
Several case studies have highlighted the effects of Vitamin B12 deficiency on health:
- Case Study on Neuropathy : A 65-year-old male presented with peripheral neuropathy attributed to Vitamin B12 deficiency. After treatment with intramuscular injections of methylcobalamin, significant improvement in neurological symptoms was observed within weeks .
- Anemia Management : A review of patients with macrocytic anemia showed that high-dose oral Vitamin B12 supplementation (1000-2000 mcg daily) effectively corrected hematological parameters within three months .
- Cognitive Function Recovery : A clinical trial involving older adults with cognitive impairment demonstrated that supplementation with 500 mcg of Vitamin B12 daily improved memory function after six months .
Research Findings
Recent studies have explored the molecular mechanisms underlying Vitamin B12's biological activity:
- Gene Expression Modulation : Research indicates that Vitamin B12 influences gene expression related to cellular metabolism and neuroprotection. Upregulation of proteins involved in antioxidant defense has been observed following supplementation .
- Network Analysis : A comprehensive network analysis revealed that Vitamin B12 affects numerous proteins involved in vital biological processes such as nucleic acid metabolism and cellular stress response .
Table 2: Differentially Expressed Proteins Post-Vitamin B12 Treatment
Protein Name | Regulation Status | Function |
---|---|---|
PTBP1 | Upregulated | Involved in pre-mRNA processing |
SOD | Upregulated | Antioxidant enzyme |
DHFR | Downregulated | Folate metabolism |
Properties
IUPAC Name |
cobalt(3+);3-[(5Z,10Z,15Z)-2,7,18-tris(2-amino-2-oxoethyl)-3,13-bis(3-amino-3-oxopropyl)-17-[3-(2-hydroxypropylamino)-3-oxopropyl]-1,2,5,7,12,12,15,17-octamethyl-8,13,18,19-tetrahydro-3H-corrin-24-id-8-yl]propanamide;cyanide;hydroxide;hydrate | |
---|---|---|
Source | PubChem | |
URL | https://pubchem.ncbi.nlm.nih.gov | |
Description | Data deposited in or computed by PubChem | |
InChI |
InChI=1S/C48H73N11O8.CN.Co.2H2O/c1-23(60)22-55-38(67)16-17-45(6)29(18-35(52)64)43-48(9)47(8,21-37(54)66)28(12-15-34(51)63)40(59-48)25(3)42-46(7,20-36(53)65)26(10-13-32(49)61)30(56-42)19-31-44(4,5)27(11-14-33(50)62)39(57-31)24(2)41(45)58-43;1-2;;;/h19,23,26-29,43,60H,10-18,20-22H2,1-9H3,(H14,49,50,51,52,53,54,55,56,57,58,59,61,62,63,64,65,66,67);;;2*1H2/q;-1;+3;;/p-2 | |
Source | PubChem | |
URL | https://pubchem.ncbi.nlm.nih.gov | |
Description | Data deposited in or computed by PubChem | |
InChI Key |
CACQFZSAEXEWBL-UHFFFAOYSA-L | |
Source | PubChem | |
URL | https://pubchem.ncbi.nlm.nih.gov | |
Description | Data deposited in or computed by PubChem | |
Canonical SMILES |
CC1=C2C(C(C([N-]2)C3(C(C(C(=N3)C(=C4C(C(C(=N4)C=C5C(C(C1=N5)CCC(=O)N)(C)C)CCC(=O)N)(C)CC(=O)N)C)CCC(=O)N)(C)CC(=O)N)C)CC(=O)N)(C)CCC(=O)NCC(C)O.[C-]#N.O.[OH-].[Co+3] | |
Source | PubChem | |
URL | https://pubchem.ncbi.nlm.nih.gov | |
Description | Data deposited in or computed by PubChem | |
Isomeric SMILES |
C/C/1=C/2\C(C(C([N-]2)C3(C(C(C(=N3)/C(=C\4/C(C(C(=N4)/C=C\5/C(C(C1=N5)CCC(=O)N)(C)C)CCC(=O)N)(C)CC(=O)N)/C)CCC(=O)N)(C)CC(=O)N)C)CC(=O)N)(C)CCC(=O)NCC(C)O.[C-]#N.O.[OH-].[Co+3] | |
Source | PubChem | |
URL | https://pubchem.ncbi.nlm.nih.gov | |
Description | Data deposited in or computed by PubChem | |
Molecular Formula |
C49H75CoN12O10 | |
Source | PubChem | |
URL | https://pubchem.ncbi.nlm.nih.gov | |
Description | Data deposited in or computed by PubChem | |
Molecular Weight |
1051.1 g/mol | |
Source | PubChem | |
URL | https://pubchem.ncbi.nlm.nih.gov | |
Description | Data deposited in or computed by PubChem | |
CAS No. |
13963-62-7 | |
Record name | Vitamin B12 factor B | |
Source | ChemIDplus | |
URL | https://pubchem.ncbi.nlm.nih.gov/substance/?source=chemidplus&sourceid=0013963627 | |
Description | ChemIDplus is a free, web search system that provides access to the structure and nomenclature authority files used for the identification of chemical substances cited in National Library of Medicine (NLM) databases, including the TOXNET system. | |
Retrosynthesis Analysis
AI-Powered Synthesis Planning: Our tool employs the Template_relevance Pistachio, Template_relevance Bkms_metabolic, Template_relevance Pistachio_ringbreaker, Template_relevance Reaxys, Template_relevance Reaxys_biocatalysis model, leveraging a vast database of chemical reactions to predict feasible synthetic routes.
One-Step Synthesis Focus: Specifically designed for one-step synthesis, it provides concise and direct routes for your target compounds, streamlining the synthesis process.
Accurate Predictions: Utilizing the extensive PISTACHIO, BKMS_METABOLIC, PISTACHIO_RINGBREAKER, REAXYS, REAXYS_BIOCATALYSIS database, our tool offers high-accuracy predictions, reflecting the latest in chemical research and data.
Strategy Settings
Precursor scoring | Relevance Heuristic |
---|---|
Min. plausibility | 0.01 |
Model | Template_relevance |
Template Set | Pistachio/Bkms_metabolic/Pistachio_ringbreaker/Reaxys/Reaxys_biocatalysis |
Top-N result to add to graph | 6 |
Feasible Synthetic Routes
Disclaimer and Information on In-Vitro Research Products
Please be aware that all articles and product information presented on BenchChem are intended solely for informational purposes. The products available for purchase on BenchChem are specifically designed for in-vitro studies, which are conducted outside of living organisms. In-vitro studies, derived from the Latin term "in glass," involve experiments performed in controlled laboratory settings using cells or tissues. It is important to note that these products are not categorized as medicines or drugs, and they have not received approval from the FDA for the prevention, treatment, or cure of any medical condition, ailment, or disease. We must emphasize that any form of bodily introduction of these products into humans or animals is strictly prohibited by law. It is essential to adhere to these guidelines to ensure compliance with legal and ethical standards in research and experimentation.