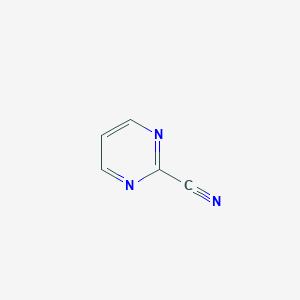
2-Cyanopyrimidine
Overview
Description
2-Cyanopyrimidine, commonly known by its brand name Pravachol, is a statin medication primarily used to prevent cardiovascular disease and treat abnormal lipid levels. It works by inhibiting the enzyme HMG-CoA reductase, which plays a crucial role in the production of cholesterol in the liver . This compound is taken orally and is often prescribed alongside lifestyle changes such as diet, exercise, and weight loss .
Mechanism of Action
Target of Action
2-Cyanopyrimidine, also known as Provastatin, primarily targets the cysteine protease cathepsin K . This enzyme plays a crucial role in various biological processes, including bone resorption and tumor progression .
On the other hand, Provastatin is a specific inhibitor of hepatic HMG-CoA reductase in humans . This enzyme plays a key role in cholesterol biosynthesis, making it a primary target for cholesterol-lowering drugs .
Mode of Action
This compound acts as a potent and non-selective inhibitor of cysteine protease cathepsin K, with an IC50 of 170 nM . By inhibiting this enzyme, this compound can effectively reduce the activity of cathepsin K, thereby influencing the processes it is involved in .
Provastatin, on the other hand, works by competitively inhibiting HMG-CoA reductase . This inhibition reduces cholesterol biosynthesis, leading to an increase in the number of LDL receptors and a subsequent decrease in plasma LDL cholesterol levels .
Biochemical Pathways
Given its role as a cathepsin k inhibitor, it is likely to affect pathways related to bone resorption and tumor progression .
Provastatin affects the cholesterol biosynthesis pathway. By inhibiting HMG-CoA reductase, it reduces the conversion of HMG-CoA to mevalonate, a key step in cholesterol synthesis . This leads to a decrease in intracellular cholesterol, inducing the expression of LDL receptors and increasing the uptake of LDL from the blood .
Pharmacokinetics
It is known that the compound’s degree of lipophilicity allows it to diffuse easily into cells .
Provastatin is rapidly absorbed after oral administration, with a bioavailability of 17% . It undergoes minimal metabolism in the liver and is eliminated through both hepatic and renal routes . The half-life of pravastatin is relatively short, less than 5 hours .
Result of Action
The molecular and cellular effects of this compound’s action are largely dependent on its inhibition of cathepsin K. By reducing the activity of this enzyme, this compound can potentially influence various biological processes, including bone resorption and tumor progression .
Provastatin’s action results in a significant reduction in plasma LDL cholesterol levels, which can help prevent cardiovascular disease .
Biochemical Analysis
Biochemical Properties
2-Cyanopyrimidine interacts with various enzymes, proteins, and other biomolecules . The nature of these interactions is complex and multifaceted, contributing to the compound’s role in biochemical reactions .
Cellular Effects
This compound has profound effects on various types of cells and cellular processes . It influences cell function, including impacts on cell signaling pathways, gene expression, and cellular metabolism .
Molecular Mechanism
The mechanism of action of this compound is intricate. It exerts its effects at the molecular level, including binding interactions with biomolecules, enzyme inhibition or activation, and changes in gene expression .
Temporal Effects in Laboratory Settings
Over time, the effects of this compound can change in laboratory settings . This includes information on the product’s stability, degradation, and any long-term effects on cellular function observed in in vitro or in vivo studies .
Dosage Effects in Animal Models
The effects of this compound vary with different dosages in animal models . This includes any threshold effects observed in these studies, as well as any toxic or adverse effects at high doses .
Metabolic Pathways
This compound is involved in various metabolic pathways . This includes interactions with enzymes or cofactors, and effects on metabolic flux or metabolite levels .
Transport and Distribution
This compound is transported and distributed within cells and tissues . This includes interactions with transporters or binding proteins, and effects on its localization or accumulation .
Subcellular Localization
The subcellular localization of this compound and its effects on activity or function are complex . This includes any targeting signals or post-translational modifications that direct it to specific compartments or organelles .
Preparation Methods
2-Cyanopyrimidine is synthesized through a combination of fermentation and chemical processes. Initially, mevastatin is obtained through the fermentation of Penicillium citrinum. This is followed by hydrolysis of the lactone group and biological hydroxylation using Streptomyces carbophilus to introduce the allylic 6-alcohol group . Industrial production involves optimizing these processes to ensure high yield and purity.
Chemical Reactions Analysis
2-Cyanopyrimidine undergoes several types of chemical reactions, including oxidation, reduction, and substitution. Common reagents used in these reactions include acids, bases, and various oxidizing and reducing agents. For instance, the electrochemical reduction of provastatin has been studied using cyclic and square-wave voltammetry, revealing that the reduction proceeds via a stable intermediate . Major products formed from these reactions include various metabolites such as 3-alpha-isomer of provastatin and a glutathione conjugate .
Scientific Research Applications
2-Cyanopyrimidine has a wide range of scientific research applications. In medicine, it is used to lower lipid levels and reduce the risk of cardiovascular events such as myocardial infarction and stroke . In biology, provastatin is studied for its effects on endothelial function, plaque stability, and thrombus formation . Additionally, it has applications in the development of nanogel-based drug delivery systems for hyperlipidemia management . In the pharmaceutical industry, provastatin is used as a model compound for studying the pharmacokinetics and pharmacodynamics of statins .
Comparison with Similar Compounds
2-Cyanopyrimidine is often compared with other statins such as atorvastatin, simvastatin, and rosuvastatin. While all these compounds inhibit HMG-CoA reductase, they differ in their potency, side effects, and pharmacokinetic profiles . For example, atorvastatin and rosuvastatin are more potent than provastatin but may have a higher incidence of muscle-related side effects . Simvastatin, on the other hand, is similar in effectiveness to provastatin but may interact with more medications . This compound is unique in its relatively lower risk of drug interactions and its hydrophilic nature, which reduces its penetration into muscle tissues and thus lowers the risk of muscle-related side effects .
Properties
IUPAC Name |
pyrimidine-2-carbonitrile | |
---|---|---|
Source | PubChem | |
URL | https://pubchem.ncbi.nlm.nih.gov | |
Description | Data deposited in or computed by PubChem | |
InChI |
InChI=1S/C5H3N3/c6-4-5-7-2-1-3-8-5/h1-3H | |
Source | PubChem | |
URL | https://pubchem.ncbi.nlm.nih.gov | |
Description | Data deposited in or computed by PubChem | |
InChI Key |
IIHQNAXFIODVDU-UHFFFAOYSA-N | |
Source | PubChem | |
URL | https://pubchem.ncbi.nlm.nih.gov | |
Description | Data deposited in or computed by PubChem | |
Canonical SMILES |
C1=CN=C(N=C1)C#N | |
Source | PubChem | |
URL | https://pubchem.ncbi.nlm.nih.gov | |
Description | Data deposited in or computed by PubChem | |
Molecular Formula |
C5H3N3 | |
Source | PubChem | |
URL | https://pubchem.ncbi.nlm.nih.gov | |
Description | Data deposited in or computed by PubChem | |
DSSTOX Substance ID |
DTXSID40864466 | |
Record name | Pyrimidine-2-carbonitrile | |
Source | EPA DSSTox | |
URL | https://comptox.epa.gov/dashboard/DTXSID40864466 | |
Description | DSSTox provides a high quality public chemistry resource for supporting improved predictive toxicology. | |
Molecular Weight |
105.10 g/mol | |
Source | PubChem | |
URL | https://pubchem.ncbi.nlm.nih.gov | |
Description | Data deposited in or computed by PubChem | |
CAS No. |
14080-23-0 | |
Record name | 2-Pyrimidinecarbonitrile | |
Source | CAS Common Chemistry | |
URL | https://commonchemistry.cas.org/detail?cas_rn=14080-23-0 | |
Description | CAS Common Chemistry is an open community resource for accessing chemical information. Nearly 500,000 chemical substances from CAS REGISTRY cover areas of community interest, including common and frequently regulated chemicals, and those relevant to high school and undergraduate chemistry classes. This chemical information, curated by our expert scientists, is provided in alignment with our mission as a division of the American Chemical Society. | |
Explanation | The data from CAS Common Chemistry is provided under a CC-BY-NC 4.0 license, unless otherwise stated. | |
Record name | Pyrimidine-2-carbonitrile | |
Source | EPA DSSTox | |
URL | https://comptox.epa.gov/dashboard/DTXSID40864466 | |
Description | DSSTox provides a high quality public chemistry resource for supporting improved predictive toxicology. | |
Record name | 2-Pyrimidinecarbonitrile | |
Source | European Chemicals Agency (ECHA) | |
URL | https://echa.europa.eu/substance-information/-/substanceinfo/100.120.209 | |
Description | The European Chemicals Agency (ECHA) is an agency of the European Union which is the driving force among regulatory authorities in implementing the EU's groundbreaking chemicals legislation for the benefit of human health and the environment as well as for innovation and competitiveness. | |
Explanation | Use of the information, documents and data from the ECHA website is subject to the terms and conditions of this Legal Notice, and subject to other binding limitations provided for under applicable law, the information, documents and data made available on the ECHA website may be reproduced, distributed and/or used, totally or in part, for non-commercial purposes provided that ECHA is acknowledged as the source: "Source: European Chemicals Agency, http://echa.europa.eu/". Such acknowledgement must be included in each copy of the material. ECHA permits and encourages organisations and individuals to create links to the ECHA website under the following cumulative conditions: Links can only be made to webpages that provide a link to the Legal Notice page. | |
Retrosynthesis Analysis
AI-Powered Synthesis Planning: Our tool employs the Template_relevance Pistachio, Template_relevance Bkms_metabolic, Template_relevance Pistachio_ringbreaker, Template_relevance Reaxys, Template_relevance Reaxys_biocatalysis model, leveraging a vast database of chemical reactions to predict feasible synthetic routes.
One-Step Synthesis Focus: Specifically designed for one-step synthesis, it provides concise and direct routes for your target compounds, streamlining the synthesis process.
Accurate Predictions: Utilizing the extensive PISTACHIO, BKMS_METABOLIC, PISTACHIO_RINGBREAKER, REAXYS, REAXYS_BIOCATALYSIS database, our tool offers high-accuracy predictions, reflecting the latest in chemical research and data.
Strategy Settings
Precursor scoring | Relevance Heuristic |
---|---|
Min. plausibility | 0.01 |
Model | Template_relevance |
Template Set | Pistachio/Bkms_metabolic/Pistachio_ringbreaker/Reaxys/Reaxys_biocatalysis |
Top-N result to add to graph | 6 |
Feasible Synthetic Routes
Disclaimer and Information on In-Vitro Research Products
Please be aware that all articles and product information presented on BenchChem are intended solely for informational purposes. The products available for purchase on BenchChem are specifically designed for in-vitro studies, which are conducted outside of living organisms. In-vitro studies, derived from the Latin term "in glass," involve experiments performed in controlled laboratory settings using cells or tissues. It is important to note that these products are not categorized as medicines or drugs, and they have not received approval from the FDA for the prevention, treatment, or cure of any medical condition, ailment, or disease. We must emphasize that any form of bodily introduction of these products into humans or animals is strictly prohibited by law. It is essential to adhere to these guidelines to ensure compliance with legal and ethical standards in research and experimentation.