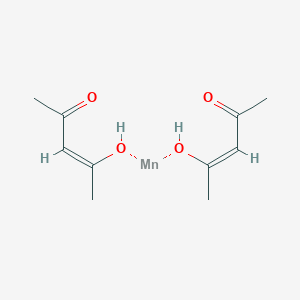
Manganese(II) acetylacetonate
Overview
Description
Manganese(II) acetylacetonate (Mn(acac)₂) is a coordination complex where manganese in the +2 oxidation state is chelated by two acetylacetonate (acac) ligands. This compound is widely utilized in materials science, catalysis, and biomedical applications due to its thermal stability, solubility in organic solvents, and redox activity. It serves as a precursor for synthesizing manganese oxide nanoparticles , ferrite nanocrystals , and functional alloys . Its versatility stems from its ability to decompose controllably under heat, releasing Mn²⁺ ions for tailored material synthesis .
Preparation Methods
Synthetic Routes and Reaction Conditions: Manganese(II) acetylacetonate can be synthesized by treating a manganese salt with acetylacetone. The general reaction involves the treatment of manganese chloride with acetylacetone in the presence of a base to facilitate the removal of a proton from acetylacetone, shifting the equilibrium in favor of the complex formation . The reaction can be represented as: [ \text{Mn}^{2+} + 2 \text{Hacac} \rightarrow \text{Mn(acac)}_2 + 2 \text{H}^+ ]
Industrial Production Methods: In industrial settings, this compound is often produced by reacting manganese chloride with acetylacetone in an ethanol solution, followed by filtration and recrystallization to purify the product . The process ensures high purity and yield, making it suitable for large-scale production.
Chemical Reactions Analysis
Types of Reactions: Manganese(II) acetylacetonate undergoes various chemical reactions, including:
Oxidation: It can be oxidized to manganese(III) acetylacetonate using oxidizing agents like potassium permanganate.
Reduction: It can be reduced to lower oxidation states under specific conditions.
Substitution: The acetylacetonate ligands can be substituted with other ligands in the presence of suitable reagents.
Common Reagents and Conditions:
Oxidation: Potassium permanganate is commonly used as an oxidizing agent.
Substitution: Various ligands can be introduced under controlled conditions to replace the acetylacetonate ligands.
Major Products:
Oxidation: Manganese(III) acetylacetonate.
Substitution: Complexes with different ligands depending on the reagents used.
Scientific Research Applications
Catalysis
Manganese(II) acetylacetonate serves as an essential catalyst in organic synthesis. Its ability to form chelate complexes enhances its reactivity, making it suitable for various catalytic processes.
- Epoxidation Reactions : A notable application is in the epoxidation of alkenes. This compound has been immobilized onto metal-organic frameworks, enhancing its catalytic efficiency for epoxidation reactions under mild conditions. This method provides a sustainable approach to synthesizing valuable epoxides from alkenes .
- Nanoparticle Synthesis : The compound is used as a precursor for synthesizing manganese oxide nanoparticles through thermal decomposition. These nanoparticles exhibit catalytic properties beneficial for various reactions, including oxidation processes and environmental remediation .
Nanomaterials Synthesis
This compound is instrumental in the production of manganese oxide nanoparticles, which have applications across multiple domains:
- Magnetic Resonance Imaging (MRI) : Manganese oxide nanoparticles synthesized from this compound are explored as contrast agents in MRI. Their tunable magnetic properties enhance imaging quality and provide better diagnostic capabilities .
- Carbon Nanostructures : Research indicates that this compound can facilitate the formation of various carbon nanostructures through chemical vapor deposition techniques. These structures have potential applications in electronics and materials science .
Membrane Technology
Recent studies highlight the use of this compound in modifying membrane properties for enhanced performance:
- ZIF-8 Membrane Modification : Vapor-phase treatment of ZIF-8 membranes with this compound improves their permselectivity, making them more efficient for gas separation processes. This advancement has significant implications for industrial applications, including carbon capture and hydrogen purification .
Environmental Applications
The compound's properties make it suitable for environmental remediation strategies:
- Wastewater Treatment : this compound has been investigated for its role in wastewater treatment processes, particularly in the removal of contaminants through catalytic oxidation reactions .
Case Study 1: Synthesis of Manganese Oxide Nanoparticles
A study demonstrated the synthesis of manganese oxide nanoparticles via thermal decomposition of this compound. The resulting nanoparticles showed promise for use in catalysis and biomedical applications due to their size-dependent properties .
Case Study 2: MRI Contrast Agents
Research conducted on manganese oxide nanoparticles derived from this compound revealed their effectiveness as T1-weighted MRI contrast agents. The study emphasized the importance of nanoparticle size and surface modification in optimizing imaging performance .
Mechanism of Action
The mechanism by which manganese(II) acetylacetonate exerts its effects involves the coordination of the acetylacetonate ligands to the manganese ion, forming a stable chelate ring. This coordination influences the reactivity and stability of the compound, making it effective in various catalytic processes . The molecular targets and pathways involved depend on the specific application and reaction conditions.
Comparison with Similar Compounds
Structural and Electronic Properties
- Manganese(III) Acetylacetonate (Mn(acac)₃) : Unlike Mn(acac)₂, Mn(acac)₃ contains Mn³⁺, resulting in distinct coordination geometry. Mn(acac)₃ adopts a trigonal-prismatic structure with six oxygen atoms from three acac ligands, while Mn(acac)₂ has a tetrahedral or octahedral geometry . The higher oxidation state of Mn³⁺ in Mn(acac)₃ enhances its oxidative catalytic activity, as seen in alkyd paint drying agents .
- Iron(III) Acetylacetonate (Fe(acac)₃): Fe(acac)₃ shares a similar trigonal geometry but exhibits a larger decomposition temperature gap (Δ >60°C) compared to Mn(acac)₂, complicating co-synthesis of mixed-metal oxides . Replacing acac with benzoylacetonate ligands reduces this gap to 13°C, improving compositional control in nanoparticle synthesis .
- Cobalt(II) Acetylacetonate (Co(acac)₂) : Co(acac)₂ shows higher biological activity than Mn(acac)₂, with Co²⁺ being ~20× more potent in vasopressin potentiation . Structurally, Co(acac)₂ forms octahedral complexes, influencing its magnetic properties in coordination assemblies .
Table 1: Structural Comparison of Metal Acetylacetonates
Catalytic Performance
- Mn(acac)₂ vs. Mn(acac)₃ : Mn(acac)₃ outperforms Mn(acac)₂ in radical-based reactions, such as 1,2-dioxane ring formation, due to its higher oxidation state enabling efficient peroxide bond formation. However, Mn(acac)₂ with Mn²⁺/Mn³⁺ acetate mixtures achieves moderate yields (30–36%) under optimized conditions .
- Cobalt-Based Catalysts : Co(acac)₂ is a benchmark drier in alkyd paints, but Mn(acac)₃ dissolved in DMSO shows comparable activity with lower toxicity, making it a sustainable alternative .
Table 3: Catalytic Efficiency in Key Reactions
Reaction | Catalyst | Yield/Activity | Reference |
---|---|---|---|
1,2-Dioxane Synthesis | Mn(acac)₃ | 36% (room temp.) | |
Alkyd Paint Drying | Mn(acac)₃/DMSO | Comparable to Co²⁺ | |
Oxidation of Alcohols | Mn(acac)₂/SiO₂-Fe | 85–92% yield |
Q & A
Basic Research Questions
Q. What are the established synthesis protocols for manganese(II) acetylacetonate, and how do reaction conditions influence product purity?
this compound is synthesized via comproportionation of Mn(acac)₂ with KMnO₄ in the presence of acetylacetone or direct reaction of acetylacetone with KMnO₄ . Purity depends on solvent choice (e.g., hydrocarbon solvents stabilize trimeric structures), reaction stoichiometry, and post-synthetic purification (e.g., recrystallization). Thermal decomposition methods (e.g., heating at 200–300°C under inert gas) yield manganese oxide nanoparticles but require precise control of ramp rates to avoid impurity phases like Mn₃O₄ .
Q. How is the structural and electronic configuration of this compound characterized?
The compound adopts a distorted octahedral geometry in the solid state, with Mn-O bond strengths of ~65 kcal/mol. Magnetic susceptibility measurements confirm a high-spin d⁵ configuration (S = 5/2). Techniques include:
- X-ray diffraction (XRD) for crystallographic analysis .
- X-ray photoelectron spectroscopy (XPS) to verify oxidation state (Mn²⁺) .
- Electron paramagnetic resonance (EPR) to study spin states and ligand field effects .
Advanced Research Questions
Q. What methodological considerations are critical when using this compound as a precursor for mixed-metal oxide electrodes?
In RuOx/MnOx electrode synthesis, precursor compatibility and decomposition kinetics are key. Mn(acac)₂ decomposes at lower temperatures (~250°C) than Ru precursors, requiring staged heating to ensure homogeneous oxide formation. Electrochemical performance (e.g., capacitance) is optimized by adjusting precursor ratios and annealing atmospheres (e.g., O₂ vs. N₂) to balance Mn³⁺/Mn⁴+ redox activity .
Q. How can core-shell MnOx nanoparticles be synthesized using this compound, and what parameters control shell thickness?
Core-shell structures (e.g., FeOx/MnOx) are synthesized via seed-mediated thermolysis:
- Step 1: Synthesize Fe₃O₄ seeds via iron oleate decomposition.
- Step 2: Deposit MnOx shells by thermolyzing Mn(acac)₂ at 300°C in the presence of seeds. Shell thickness (1–5 nm) is tuned by varying precursor concentration, reaction time, and surfactant (e.g., oleylamine) ratios. High-resolution TEM and XANES confirm core-shell integrity and Mn oxidation states .
Q. How do researchers resolve contradictions in reported catalytic activities of Mn(acac)₂-derived materials?
Discrepancies in catalytic efficiency (e.g., for CO oxidation) arise from differences in:
- Surface defects: Annealing conditions (e.g., air vs. vacuum) alter oxygen vacancy concentrations.
- Phase purity: Unintended Mn₃O₄ impurities reduce activity, detected via XRD and Raman spectroscopy.
- Support interactions: TiO₂ vs. Al₂O₃ supports modify Mn dispersion and redox behavior. Comparative studies using in-situ DRIFTS and kinetic isotopic effect (KIE) analysis isolate active sites .
Q. What strategies improve the biocompatibility of this compound-derived nanoparticles for drug delivery?
For biomedical applications (e.g., FA-BSA-DOX MnFe₂O₄ NPs):
- Surface functionalization: Coating with bovine serum albumin (BSA) reduces cytotoxicity.
- Size control: Sub-20 nm particles (achieved via polyol synthesis with triethylene glycol) enhance tumor penetration.
- Chelation: Excess acetylacetone ligand removal (via dialysis) minimizes Mn²⁺ leaching, verified by ICP-MS .
Q. Methodological Tables
Table 1: Comparison of Synthesis Methods for Mn(acac)₂-Derived Nanoparticles
Table 2: Common Analytical Techniques for Resolving Data Contradictions
Issue | Technique | Application Example |
---|---|---|
Phase impurities | XRD/Raman | Distinguishing MnO vs. Mn₃O₄ phases |
Surface oxidation states | XPS/XANES | Quantifying Mn²⁺/Mn³⁺ ratios in catalysts |
Leaching in biomedicine | ICP-MS | Measuring Mn²⁺ release from nanoparticles |
Properties
CAS No. |
14024-58-9 |
---|---|
Molecular Formula |
C10H16MnO4 |
Molecular Weight |
255.17 g/mol |
IUPAC Name |
4-hydroxypent-3-en-2-one;manganese |
InChI |
InChI=1S/2C5H8O2.Mn/c2*1-4(6)3-5(2)7;/h2*3,6H,1-2H3; |
InChI Key |
VNNDVNZCGCCIPA-UHFFFAOYSA-N |
SMILES |
CC(=CC(=O)C)O.CC(=CC(=O)C)O.[Mn] |
Isomeric SMILES |
C/C(=C/C(=O)C)/O.C/C(=C/C(=O)C)/O.[Mn] |
Canonical SMILES |
CC(=CC(=O)C)O.CC(=CC(=O)C)O.[Mn] |
Key on ui other cas no. |
14024-58-9 |
physical_description |
Beige solid; [Sigma-Aldrich MSDS] |
Pictograms |
Irritant; Health Hazard |
Related CAS |
17272-66-1 (Parent) |
Synonyms |
acetyl acetonate acetyl acetonate, chromium (III) salt acetyl acetonate, copper (+2) salt acetyl acetonate, manganese (II) salt acetyl acetonate, manganese (III) salt acetyl acetonate, potassium salt acetyl acetonate, sodium salt acetylacetonate chromium(III) acetylacetonate copper acetylacetonate copper(II) acetylacetonate Cu(II) acetyl acetonate cupric acetylacetonate manganese acetylacetonate Nd(III)-acetylacetonate vanadyl acetylacetonate |
Origin of Product |
United States |
Retrosynthesis Analysis
AI-Powered Synthesis Planning: Our tool employs the Template_relevance Pistachio, Template_relevance Bkms_metabolic, Template_relevance Pistachio_ringbreaker, Template_relevance Reaxys, Template_relevance Reaxys_biocatalysis model, leveraging a vast database of chemical reactions to predict feasible synthetic routes.
One-Step Synthesis Focus: Specifically designed for one-step synthesis, it provides concise and direct routes for your target compounds, streamlining the synthesis process.
Accurate Predictions: Utilizing the extensive PISTACHIO, BKMS_METABOLIC, PISTACHIO_RINGBREAKER, REAXYS, REAXYS_BIOCATALYSIS database, our tool offers high-accuracy predictions, reflecting the latest in chemical research and data.
Strategy Settings
Precursor scoring | Relevance Heuristic |
---|---|
Min. plausibility | 0.01 |
Model | Template_relevance |
Template Set | Pistachio/Bkms_metabolic/Pistachio_ringbreaker/Reaxys/Reaxys_biocatalysis |
Top-N result to add to graph | 6 |
Feasible Synthetic Routes
Disclaimer and Information on In-Vitro Research Products
Please be aware that all articles and product information presented on BenchChem are intended solely for informational purposes. The products available for purchase on BenchChem are specifically designed for in-vitro studies, which are conducted outside of living organisms. In-vitro studies, derived from the Latin term "in glass," involve experiments performed in controlled laboratory settings using cells or tissues. It is important to note that these products are not categorized as medicines or drugs, and they have not received approval from the FDA for the prevention, treatment, or cure of any medical condition, ailment, or disease. We must emphasize that any form of bodily introduction of these products into humans or animals is strictly prohibited by law. It is essential to adhere to these guidelines to ensure compliance with legal and ethical standards in research and experimentation.