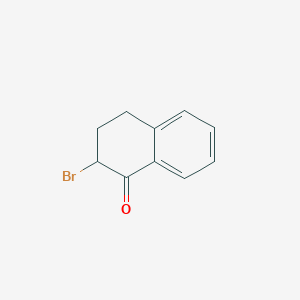
2-Bromo-1-tetralone
Overview
Description
Preparation Methods
Synthetic Routes and Reaction Conditions: 2-Bromo-1-tetralone can be synthesized through several methods. One common approach involves the bromination of 1-tetralone using bromine in the presence of a suitable solvent such as acetic acid. The reaction typically proceeds at room temperature, yielding this compound as the primary product .
Industrial Production Methods: In an industrial setting, the production of this compound may involve continuous flow processes to ensure high yield and purity. The use of advanced bromination techniques and optimized reaction conditions can enhance the efficiency of the production process .
Chemical Reactions Analysis
Types of Reactions: 2-Bromo-1-tetralone undergoes various chemical reactions, including:
Substitution Reactions: The bromine atom can be substituted with other functional groups using nucleophilic substitution reactions.
Reduction Reactions: The carbonyl group in this compound can be reduced to form corresponding alcohols.
Oxidation Reactions: The compound can be oxidized to form carboxylic acids or other oxidized derivatives.
Common Reagents and Conditions:
Substitution: Sodium hydroxide or potassium hydroxide in an aqueous or alcoholic medium.
Reduction: Lithium aluminum hydride or sodium borohydride in anhydrous ether.
Oxidation: Potassium permanganate or chromium trioxide in acidic conditions.
Major Products:
Substitution: Various substituted tetralones depending on the nucleophile used.
Reduction: 2-Bromo-1-tetralol.
Oxidation: this compound carboxylic acid.
Scientific Research Applications
2-Bromo-1-tetralone has a wide range of applications in scientific research:
Chemistry: It serves as a key intermediate in the synthesis of various heterocyclic compounds and pharmaceuticals.
Biology: The compound is used in the study of enzyme inhibition and as a probe in biochemical assays.
Industry: this compound is utilized in the production of agrochemicals and other industrial chemicals.
Mechanism of Action
The mechanism of action of 2-Bromo-1-tetralone involves its interaction with specific molecular targets. For instance, in medicinal chemistry, it may act as an inhibitor of certain enzymes by binding to their active sites. This interaction can modulate the activity of the enzymes, leading to therapeutic effects. The bromine atom and the carbonyl group play crucial roles in its binding affinity and reactivity .
Comparison with Similar Compounds
1-Tetralone: Lacks the bromine atom, making it less reactive in certain substitution reactions.
2-Chloro-1-tetralone: Similar structure but with a chlorine atom instead of bromine, leading to different reactivity and applications.
2-Iodo-1-tetralone: Contains an iodine atom, which can influence its reactivity and use in organic synthesis.
Uniqueness: 2-Bromo-1-tetralone is unique due to the presence of the bromine atom, which enhances its reactivity in substitution reactions. This makes it a versatile intermediate in the synthesis of various complex molecules .
Properties
IUPAC Name |
2-bromo-3,4-dihydro-2H-naphthalen-1-one | |
---|---|---|
Source | PubChem | |
URL | https://pubchem.ncbi.nlm.nih.gov | |
Description | Data deposited in or computed by PubChem | |
InChI |
InChI=1S/C10H9BrO/c11-9-6-5-7-3-1-2-4-8(7)10(9)12/h1-4,9H,5-6H2 | |
Source | PubChem | |
URL | https://pubchem.ncbi.nlm.nih.gov | |
Description | Data deposited in or computed by PubChem | |
InChI Key |
AYNCWMIFKFADCZ-UHFFFAOYSA-N | |
Source | PubChem | |
URL | https://pubchem.ncbi.nlm.nih.gov | |
Description | Data deposited in or computed by PubChem | |
Canonical SMILES |
C1CC2=CC=CC=C2C(=O)C1Br | |
Source | PubChem | |
URL | https://pubchem.ncbi.nlm.nih.gov | |
Description | Data deposited in or computed by PubChem | |
Molecular Formula |
C10H9BrO | |
Source | PubChem | |
URL | https://pubchem.ncbi.nlm.nih.gov | |
Description | Data deposited in or computed by PubChem | |
DSSTOX Substance ID |
DTXSID40884629 | |
Record name | 1(2H)-Naphthalenone, 2-bromo-3,4-dihydro- | |
Source | EPA DSSTox | |
URL | https://comptox.epa.gov/dashboard/DTXSID40884629 | |
Description | DSSTox provides a high quality public chemistry resource for supporting improved predictive toxicology. | |
Molecular Weight |
225.08 g/mol | |
Source | PubChem | |
URL | https://pubchem.ncbi.nlm.nih.gov | |
Description | Data deposited in or computed by PubChem | |
CAS No. |
13672-07-6 | |
Record name | 2-Bromo-1-tetralone | |
Source | CAS Common Chemistry | |
URL | https://commonchemistry.cas.org/detail?cas_rn=13672-07-6 | |
Description | CAS Common Chemistry is an open community resource for accessing chemical information. Nearly 500,000 chemical substances from CAS REGISTRY cover areas of community interest, including common and frequently regulated chemicals, and those relevant to high school and undergraduate chemistry classes. This chemical information, curated by our expert scientists, is provided in alignment with our mission as a division of the American Chemical Society. | |
Explanation | The data from CAS Common Chemistry is provided under a CC-BY-NC 4.0 license, unless otherwise stated. | |
Record name | 1(2H)-Naphthalenone, 2-bromo-3,4-dihydro- | |
Source | ChemIDplus | |
URL | https://pubchem.ncbi.nlm.nih.gov/substance/?source=chemidplus&sourceid=0013672076 | |
Description | ChemIDplus is a free, web search system that provides access to the structure and nomenclature authority files used for the identification of chemical substances cited in National Library of Medicine (NLM) databases, including the TOXNET system. | |
Record name | 2-Bromo-1-tetralone | |
Source | DTP/NCI | |
URL | https://dtp.cancer.gov/dtpstandard/servlet/dwindex?searchtype=NSC&outputformat=html&searchlist=402212 | |
Description | The NCI Development Therapeutics Program (DTP) provides services and resources to the academic and private-sector research communities worldwide to facilitate the discovery and development of new cancer therapeutic agents. | |
Explanation | Unless otherwise indicated, all text within NCI products is free of copyright and may be reused without our permission. Credit the National Cancer Institute as the source. | |
Record name | 1(2H)-Naphthalenone, 2-bromo-3,4-dihydro- | |
Source | EPA Chemicals under the TSCA | |
URL | https://www.epa.gov/chemicals-under-tsca | |
Description | EPA Chemicals under the Toxic Substances Control Act (TSCA) collection contains information on chemicals and their regulations under TSCA, including non-confidential content from the TSCA Chemical Substance Inventory and Chemical Data Reporting. | |
Record name | 1(2H)-Naphthalenone, 2-bromo-3,4-dihydro- | |
Source | EPA DSSTox | |
URL | https://comptox.epa.gov/dashboard/DTXSID40884629 | |
Description | DSSTox provides a high quality public chemistry resource for supporting improved predictive toxicology. | |
Record name | 2-bromo-3,4-dihydronaphthalen-1(2H)-one | |
Source | European Chemicals Agency (ECHA) | |
URL | https://echa.europa.eu/substance-information/-/substanceinfo/100.033.762 | |
Description | The European Chemicals Agency (ECHA) is an agency of the European Union which is the driving force among regulatory authorities in implementing the EU's groundbreaking chemicals legislation for the benefit of human health and the environment as well as for innovation and competitiveness. | |
Explanation | Use of the information, documents and data from the ECHA website is subject to the terms and conditions of this Legal Notice, and subject to other binding limitations provided for under applicable law, the information, documents and data made available on the ECHA website may be reproduced, distributed and/or used, totally or in part, for non-commercial purposes provided that ECHA is acknowledged as the source: "Source: European Chemicals Agency, http://echa.europa.eu/". Such acknowledgement must be included in each copy of the material. ECHA permits and encourages organisations and individuals to create links to the ECHA website under the following cumulative conditions: Links can only be made to webpages that provide a link to the Legal Notice page. | |
Retrosynthesis Analysis
AI-Powered Synthesis Planning: Our tool employs the Template_relevance Pistachio, Template_relevance Bkms_metabolic, Template_relevance Pistachio_ringbreaker, Template_relevance Reaxys, Template_relevance Reaxys_biocatalysis model, leveraging a vast database of chemical reactions to predict feasible synthetic routes.
One-Step Synthesis Focus: Specifically designed for one-step synthesis, it provides concise and direct routes for your target compounds, streamlining the synthesis process.
Accurate Predictions: Utilizing the extensive PISTACHIO, BKMS_METABOLIC, PISTACHIO_RINGBREAKER, REAXYS, REAXYS_BIOCATALYSIS database, our tool offers high-accuracy predictions, reflecting the latest in chemical research and data.
Strategy Settings
Precursor scoring | Relevance Heuristic |
---|---|
Min. plausibility | 0.01 |
Model | Template_relevance |
Template Set | Pistachio/Bkms_metabolic/Pistachio_ringbreaker/Reaxys/Reaxys_biocatalysis |
Top-N result to add to graph | 6 |
Feasible Synthetic Routes
Q1: What are the common synthetic applications of 2-bromo-1-tetralone?
A: this compound serves as a versatile building block in organic synthesis. One notable application is its reaction with arylthioacetamides, leading to the formation of indeno- and naphtho-fused thiophenes. [] These fused heterocyclic compounds hold potential in materials science and medicinal chemistry due to their unique properties.
Q2: How does this compound react with cyanogen bromide?
A: Interestingly, this compound doesn't react with cyanogen bromide in the same manner as 2-tetralone. While 2-tetralone undergoes aromatization to form naphthols and naphthylamines upon reaction with cyanogen bromide, this compound remains unreactive under similar conditions. [] This difference in reactivity highlights the influence of the bromine substituent on the chemical behavior of the tetralone scaffold.
Q3: Are there any studies on the reactions of this compound with phenols?
A: While specific details about the reaction outcomes are not available in the provided abstracts, research exists exploring the reactions between this compound and phenols. [] These reactions likely involve nucleophilic substitution or addition reactions, potentially yielding diverse and complex molecular structures. Further investigation into these reactions could provide valuable insights into the reactivity profile of this compound and pave the way for novel synthetic applications.
Disclaimer and Information on In-Vitro Research Products
Please be aware that all articles and product information presented on BenchChem are intended solely for informational purposes. The products available for purchase on BenchChem are specifically designed for in-vitro studies, which are conducted outside of living organisms. In-vitro studies, derived from the Latin term "in glass," involve experiments performed in controlled laboratory settings using cells or tissues. It is important to note that these products are not categorized as medicines or drugs, and they have not received approval from the FDA for the prevention, treatment, or cure of any medical condition, ailment, or disease. We must emphasize that any form of bodily introduction of these products into humans or animals is strictly prohibited by law. It is essential to adhere to these guidelines to ensure compliance with legal and ethical standards in research and experimentation.