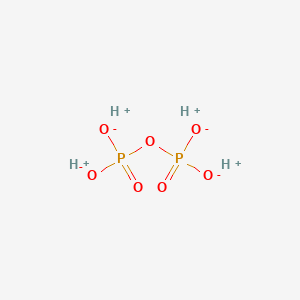
Pyrophosphate ion
Overview
Description
Pyrophosphates are phosphorus oxyanions that contain two phosphorus atoms in a P−O−P linkage . A number of pyrophosphate salts exist, such as disodium pyrophosphate (Na2H2P2O7) and tetrasodium pyrophosphate (Na4P2O7), among others . Often pyrophosphates are called diphosphates . The parent pyrophosphates are derived from partial or complete neutralization of pyrophosphoric acid . The pyrophosphate bond is also sometimes referred to as a phosphoanhydride bond . Pyrophosphates are found in ATP and other nucleotide triphosphates, which are important in biochemistry .
Synthesis Analysis
Disodium pyrophosphate is prepared by thermal condensation of sodium dihydrogen phosphate or by partial deprotonation of pyrophosphoric acid . Pyrophosphates are generally white or colorless . The alkali metal salts are water-soluble . They are good complexing agents for metal ions (such as calcium and many transition metals) and have many uses in industrial chemistry .
Molecular Structure Analysis
The pyrophosphate anion has the structure P2O7^4- , and is an acid anhydride of phosphate . It is unstable in aqueous solution and rapidly hydrolyzes into inorganic phosphate .
Chemical Reactions Analysis
Quantitative analysis of five pyrophosphates, including isopentenyl pyrophosphate (IPP), dimethylallyl pyrophosphate (DMAPP), geranyl pyrophosphate (GPP), farnesyl pyrophosphate (FPP), and geranylgeranyl pyrophosphate (GGPP), was performed using liquid chromatography-tandem mass spectrometry (LC-MS/MS) in negative ionization mode .
Physical And Chemical Properties Analysis
Pyrophosphoric acid is a tetraprotic acid, with four distinct pKa’s . The pKa’s occur in two distinct ranges because deprotonations occur on separate phosphate groups . At physiological pH’s, pyrophosphate exists as a mixture of doubly and singly protonated forms . Pyrophosphates are generally white or colorless . The alkali metal salts are water-soluble .
Scientific Research Applications
Soil Science and Agriculture : PPi is a significant component in fluid fertilizers like ammonium polyphosphate (APP). When APP is applied to soil, PPi is hydrolyzed to orthophosphate, influencing the availability of phosphorus in the soil. This process and its implications for phosphorus availability are currently under investigation in Australian soil types (McBeath et al., 2006).
Biochemistry and Molecular Biology : The selective detection of PPi is crucial as it is the product of ATP hydrolysis and involved in DNA replication and cancer research. Various methods, including fluorescent chemosensors and colorimetric sensors, have been developed to detect PPi in biological systems (Kim et al., 2009).
Materials Science : In the field of materials science, iron-based pyrophosphate compounds like Na2FeP2O7 have been explored as positive electrode materials for rechargeable aqueous sodium-ion batteries (Jung et al., 2014).
Medical Research and Diagnostics : PPi plays a role in various biological processes and is a marker in clinical diagnosis. Its presence and concentration can be indicative of certain medical conditions. For instance, fluorescent and colorimetric chemosensors have been developed for the detection of PPi, aiding in the diagnosis and understanding of various diseases (Lee et al., 2015).
Bone and Dental Health : PPi is involved in the regulation of bone mineralization and may influence bone formation. For example, pyrophosphate ions are known to inhibit hydroxyapatite formation, and their concentration and regulation have implications for bone health and the treatment of conditions like osteoporosis (Grover et al., 2013).
Vascular Health : Treatment with pyrophosphate has been shown to inhibit vascular calcification, which is particularly relevant in the context of advanced renal failure and related conditions (O'Neill et al., 2011).
Mechanism of Action
Safety and Hazards
properties
IUPAC Name |
phosphonato phosphate | |
---|---|---|
Source | PubChem | |
URL | https://pubchem.ncbi.nlm.nih.gov | |
Description | Data deposited in or computed by PubChem | |
InChI |
InChI=1S/H4O7P2/c1-8(2,3)7-9(4,5)6/h(H2,1,2,3)(H2,4,5,6)/p-4 | |
Source | PubChem | |
URL | https://pubchem.ncbi.nlm.nih.gov | |
Description | Data deposited in or computed by PubChem | |
InChI Key |
XPPKVPWEQAFLFU-UHFFFAOYSA-J | |
Source | PubChem | |
URL | https://pubchem.ncbi.nlm.nih.gov | |
Description | Data deposited in or computed by PubChem | |
Canonical SMILES |
[O-]P(=O)([O-])OP(=O)([O-])[O-] | |
Source | PubChem | |
URL | https://pubchem.ncbi.nlm.nih.gov | |
Description | Data deposited in or computed by PubChem | |
Molecular Formula |
O7P2-4 | |
Source | PubChem | |
URL | https://pubchem.ncbi.nlm.nih.gov | |
Description | Data deposited in or computed by PubChem | |
DSSTOX Substance ID |
DTXSID4074514 | |
Record name | Diphosphate | |
Source | EPA DSSTox | |
URL | https://comptox.epa.gov/dashboard/DTXSID4074514 | |
Description | DSSTox provides a high quality public chemistry resource for supporting improved predictive toxicology. | |
Molecular Weight |
173.94 g/mol | |
Source | PubChem | |
URL | https://pubchem.ncbi.nlm.nih.gov | |
Description | Data deposited in or computed by PubChem | |
Product Name |
Diphosphate | |
CAS RN |
14000-31-8 | |
Record name | Pyrophosphate | |
Source | CAS Common Chemistry | |
URL | https://commonchemistry.cas.org/detail?cas_rn=14000-31-8 | |
Description | CAS Common Chemistry is an open community resource for accessing chemical information. Nearly 500,000 chemical substances from CAS REGISTRY cover areas of community interest, including common and frequently regulated chemicals, and those relevant to high school and undergraduate chemistry classes. This chemical information, curated by our expert scientists, is provided in alignment with our mission as a division of the American Chemical Society. | |
Explanation | The data from CAS Common Chemistry is provided under a CC-BY-NC 4.0 license, unless otherwise stated. | |
Record name | Pyrophosphate | |
Source | ChemIDplus | |
URL | https://pubchem.ncbi.nlm.nih.gov/substance/?source=chemidplus&sourceid=0014000318 | |
Description | ChemIDplus is a free, web search system that provides access to the structure and nomenclature authority files used for the identification of chemical substances cited in National Library of Medicine (NLM) databases, including the TOXNET system. | |
Record name | Diphosphate | |
Source | EPA DSSTox | |
URL | https://comptox.epa.gov/dashboard/DTXSID4074514 | |
Description | DSSTox provides a high quality public chemistry resource for supporting improved predictive toxicology. | |
Record name | 14000-31-8 | |
Source | European Chemicals Agency (ECHA) | |
URL | https://echa.europa.eu/information-on-chemicals | |
Description | The European Chemicals Agency (ECHA) is an agency of the European Union which is the driving force among regulatory authorities in implementing the EU's groundbreaking chemicals legislation for the benefit of human health and the environment as well as for innovation and competitiveness. | |
Explanation | Use of the information, documents and data from the ECHA website is subject to the terms and conditions of this Legal Notice, and subject to other binding limitations provided for under applicable law, the information, documents and data made available on the ECHA website may be reproduced, distributed and/or used, totally or in part, for non-commercial purposes provided that ECHA is acknowledged as the source: "Source: European Chemicals Agency, http://echa.europa.eu/". Such acknowledgement must be included in each copy of the material. ECHA permits and encourages organisations and individuals to create links to the ECHA website under the following cumulative conditions: Links can only be made to webpages that provide a link to the Legal Notice page. | |
Record name | PYROPHOSPHATE | |
Source | FDA Global Substance Registration System (GSRS) | |
URL | https://gsrs.ncats.nih.gov/ginas/app/beta/substances/X3SSV2V6L3 | |
Description | The FDA Global Substance Registration System (GSRS) enables the efficient and accurate exchange of information on what substances are in regulated products. Instead of relying on names, which vary across regulatory domains, countries, and regions, the GSRS knowledge base makes it possible for substances to be defined by standardized, scientific descriptions. | |
Explanation | Unless otherwise noted, the contents of the FDA website (www.fda.gov), both text and graphics, are not copyrighted. They are in the public domain and may be republished, reprinted and otherwise used freely by anyone without the need to obtain permission from FDA. Credit to the U.S. Food and Drug Administration as the source is appreciated but not required. | |
Retrosynthesis Analysis
AI-Powered Synthesis Planning: Our tool employs the Template_relevance Pistachio, Template_relevance Bkms_metabolic, Template_relevance Pistachio_ringbreaker, Template_relevance Reaxys, Template_relevance Reaxys_biocatalysis model, leveraging a vast database of chemical reactions to predict feasible synthetic routes.
One-Step Synthesis Focus: Specifically designed for one-step synthesis, it provides concise and direct routes for your target compounds, streamlining the synthesis process.
Accurate Predictions: Utilizing the extensive PISTACHIO, BKMS_METABOLIC, PISTACHIO_RINGBREAKER, REAXYS, REAXYS_BIOCATALYSIS database, our tool offers high-accuracy predictions, reflecting the latest in chemical research and data.
Strategy Settings
Precursor scoring | Relevance Heuristic |
---|---|
Min. plausibility | 0.01 |
Model | Template_relevance |
Template Set | Pistachio/Bkms_metabolic/Pistachio_ringbreaker/Reaxys/Reaxys_biocatalysis |
Top-N result to add to graph | 6 |
Feasible Synthetic Routes
Disclaimer and Information on In-Vitro Research Products
Please be aware that all articles and product information presented on BenchChem are intended solely for informational purposes. The products available for purchase on BenchChem are specifically designed for in-vitro studies, which are conducted outside of living organisms. In-vitro studies, derived from the Latin term "in glass," involve experiments performed in controlled laboratory settings using cells or tissues. It is important to note that these products are not categorized as medicines or drugs, and they have not received approval from the FDA for the prevention, treatment, or cure of any medical condition, ailment, or disease. We must emphasize that any form of bodily introduction of these products into humans or animals is strictly prohibited by law. It is essential to adhere to these guidelines to ensure compliance with legal and ethical standards in research and experimentation.