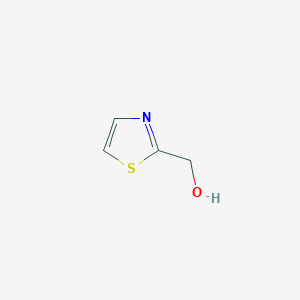
1,3-Thiazol-2-ylmethanol
Overview
Description
1,3-Thiazol-2-ylmethanol is a heterocyclic organic compound characterized by a thiazole ring (a five-membered ring containing sulfur and nitrogen) substituted with a hydroxymethyl group at the 2-position. Its molecular formula is C₄H₅NOS, with a molecular weight of 115.15 g/mol (inferred from structural analogs in ). This compound serves as a versatile intermediate in medicinal chemistry and organic synthesis, particularly in the design of pharmacologically active molecules due to the thiazole ring’s electron-rich nature and hydrogen-bonding capability .
Preparation Methods
Synthetic Routes and Reaction Conditions
1,3-Thiazol-2-ylmethanol can be synthesized from 2-bromothiazole. The synthetic route involves the conversion of 2-bromothiazole to thiazole-2-aldehyde, which is then reduced to obtain this compound . The reaction conditions typically involve the use of reducing agents such as sodium borohydride or lithium aluminum hydride under controlled temperature and pressure conditions.
Industrial Production Methods
Industrial production methods for this compound are not well-documented in the literature. the synthesis process can be scaled up by optimizing reaction conditions and using industrial-grade reagents and equipment.
Chemical Reactions Analysis
Oxidation Reactions
The hydroxymethyl group undergoes oxidation to form a carboxylic acid or ketone.
-
Reagents/Conditions :
-
Potassium permanganate (KMnO₄) in acidic or neutral media.
-
Chromium trioxide (CrO₃) under controlled conditions.
-
-
Products :
Example :
Lithiation followed by CO₂ quenching yields 1,3-thiazole-5-carboxylic acid derivatives (82% yield) .
Substitution Reactions
The thiazole ring and hydroxymethyl group participate in nucleophilic and electrophilic substitutions.
Electrophilic Substitution
-
Reagents : Acetaldehyde, cyclohexanone, or morpholin-4-carbaldehyde .
-
Conditions : Lithiation with LDA (Lithium Diisopropylamide) at -70°C, followed by electrophilic quenching .
Electrophile | Product | Yield (%) | Reference |
---|---|---|---|
Acetaldehyde | 1-(1,3-Thiazol-5-yl)ethan-1-ol | 78 | |
Cyclohexanone | 1-(1,3-Thiazol-5-yl)cyclohexan-1-ol | 65 | |
CO₂ | 1,3-Thiazole-5-carboxylic acid | 74 |
Nucleophilic Substitution
Esterification and Etherification
The hydroxymethyl group forms esters or ethers under standard conditions.
-
Esterification : Reaction with ethyl chloroacetate yields thiazolidin-4-one derivatives (e.g., compound 22 ) .
-
Etherification : Alkylation with alkyl halides in the presence of a base (e.g., K₂CO₃).
Example :
Reaction with ethyl chloroacetate:
Condensation Reactions
The alcohol group participates in dehydrative condensations.
-
With Hydrazonoyl Chlorides : Forms 1,3,4-thiadiazole derivatives (e.g., compounds 6 , 10a–e ) .
-
With Aldehydes : Produces 5-arylidene thiazolidin-4-ones (e.g., 23a–f ) .
Notable Example :
Condensation with benzaldehyde yields 5-benzylidene-thiazolidin-4-one (23a ), showing moderate anticancer activity (IC₅₀: 12–18 µM) .
Complex Formation
The thiazole nitrogen and hydroxyl group coordinate with metal ions.
Biological Activity Correlations
Derivatives exhibit structure-dependent bioactivity:
Scientific Research Applications
Biological Activities
1,3-Thiazol-2-ylmethanol has been associated with several biological activities:
- Antimicrobial Properties: Thiazole derivatives have demonstrated significant antimicrobial effects. Research indicates that compounds similar to this compound can inhibit the growth of various bacteria and fungi. For example, studies have shown effective inhibition against pathogenic strains such as C. albicans and E. coli .
- Anticancer Activity: Several studies have explored the anticancer potential of thiazole derivatives. For instance, novel thiazole compounds have been synthesized and tested against cancer cell lines (e.g., A549 lung adenocarcinoma) with promising results, indicating selective cytotoxicity . The structure-activity relationship (SAR) studies suggest that modifications to the thiazole ring can enhance anticancer efficacy .
- Enzyme Inhibition: this compound has been investigated for its ability to inhibit specific enzymes involved in metabolic pathways. For example, it has shown potential as an inhibitor of carbonic anhydrase (CA) III, which is relevant in cancer therapy .
Synthetic Applications
The compound serves as a versatile building block in organic synthesis. It can be utilized to develop more complex molecules with desired biological activities:
- Synthesis Methods: Various synthetic routes exist for producing this compound, often involving reactions with aldehydes or other thiazole derivatives .
Case Studies and Research Findings
A selection of case studies highlights the applications of this compound in scientific research:
Interaction Studies
Research has also focused on the interactions between this compound and biological targets. Techniques such as molecular docking simulations and biochemical assays are employed to assess binding affinities with enzymes and receptors relevant to disease pathways.
Mechanism of Action
The mechanism of action of 1,3-Thiazol-2-ylmethanol involves its interaction with various molecular targets and pathways. The thiazole ring’s aromaticity allows it to participate in various biochemical reactions, including:
Enzyme Inhibition: The compound can inhibit enzymes involved in metabolic pathways, leading to therapeutic effects.
Receptor Binding: It can bind to specific receptors, modulating their activity and resulting in biological effects.
Comparison with Similar Compounds
Structural Isomers: Positional Variations on Aromatic Rings
Thiazolylmethanol derivatives with aromatic substituents exhibit distinct properties based on substitution patterns. For example:
Key Differences :
- Meta vs. para substitution influences electronic distribution and steric effects. The para isomer may exhibit higher symmetry and crystallinity, impacting solubility and reactivity .
- Applications : Meta-substituted derivatives are often employed in metal-organic frameworks, while para-substituted analogs are explored for antimicrobial and anticancer activities .
Functional Group Variations in Thiazole Derivatives
Modifications to the hydroxymethyl group or thiazole ring alter physicochemical and biological properties:
Key Insights :
- Hydroxymethyl vs. acetamide groups : Hydroxymethyl enhances hydrophilicity, while acetamide groups improve metabolic stability and target binding .
- Halogenation : Chlorine substituents increase lipophilicity and membrane permeability, critical for antimicrobial activity .
Heterocyclic Analogs: Thiazole vs. Thiadiazole Derivatives
Thiadiazoles (sulfur-nitrogen heterocycles with two nitrogen atoms) differ in electronic properties and applications:
Comparison :
- Aromatic Stability : Thiadiazoles exhibit stronger aromaticity, enhancing thermal stability but reducing reactivity in electrophilic substitutions .
- Bioactivity : Thiadiazoles are more commonly reported for antitumor and enzyme inhibitory activities due to their planar structure and hydrogen-bonding capacity .
Yield and Efficiency :
- Thiosemicarbazones achieve >90% yields under mild conditions , whereas Hantzsch reactions may require longer reaction times (~6–12 hours) .
Biological Activity
1,3-Thiazol-2-ylmethanol is a compound of significant interest in medicinal chemistry due to its diverse biological activities. This article provides a comprehensive overview of its synthesis, biological properties, and potential therapeutic applications, supported by data tables and research findings.
Chemical Structure and Synthesis
This compound (C4H5NOS) features a thiazole ring, which is known for its role in various pharmacological activities. The compound can be synthesized through several methods, including the reaction of thiazole derivatives with aldehydes or via cyclization reactions involving thioketones and amines.
Antimicrobial Activity
Numerous studies have highlighted the antimicrobial properties of this compound and its derivatives. For instance, a study demonstrated that thiazole compounds exhibited potent antibacterial activity against both Gram-positive and Gram-negative bacteria. The Minimum Inhibitory Concentration (MIC) values for various bacterial strains are summarized in the following table:
Bacterial Species | MIC (μg/mL) | Gram Stain | Morphology |
---|---|---|---|
Staphylococcus aureus | 3.9 | Positive | Spherical |
Bacillus cereus | 7.8 | Positive | Rod |
Escherichia coli | Not active | Negative | Rod |
Pseudomonas aeruginosa | 7.8 | Negative | Rod |
These findings indicate that this compound has stronger antibacterial properties compared to standard antibiotics like oxytetracycline .
Anticancer Activity
Research has also explored the anticancer potential of thiazole derivatives. For example, compounds containing the thiazole moiety have been shown to inhibit cell proliferation in various cancer cell lines. A specific study reported that modifications at the 5-position of the thiazole ring significantly enhanced cytotoxicity against cancer cells. The mechanism of action often involves the induction of apoptosis and cell cycle arrest .
Case Studies
Several case studies have documented the therapeutic applications of this compound:
- Study on Antibacterial Activity : A recent investigation assessed the efficacy of synthesized thiazole derivatives against multi-drug resistant strains of bacteria. The results indicated that certain derivatives exhibited MIC values as low as 3.9 μg/mL against Staphylococcus aureus, suggesting potential for development as new antibacterial agents .
- Anticancer Research : In vitro studies demonstrated that thiazole derivatives could effectively inhibit the growth of breast cancer cells by inducing apoptosis through mitochondrial pathways. This highlights their potential as lead compounds in cancer therapy .
Pharmacological Mechanisms
The pharmacological mechanisms underlying the activities of this compound include:
- Inhibition of Enzymatic Pathways : Compounds have been identified as inhibitors of p38 MAP kinase and phosphodiesterase IV (PDE IV), which are crucial in inflammatory responses and cellular signaling pathways .
- Antioxidant Properties : Some derivatives exhibit antioxidant activity, contributing to their protective effects against oxidative stress-related diseases .
Q & A
Basic Research Questions
Q. What are the optimal synthetic routes for preparing 1,3-Thiazol-2-ylmethanol with high purity for crystallographic studies?
- Methodology : A robust synthesis involves condensation reactions between thiazole precursors and formaldehyde derivatives under controlled pH (e.g., using catalysts like Bleaching Earth Clay at pH 12.5). Purification via recrystallization in polar solvents (e.g., aqueous acetic acid) ensures high yields and purity. Reaction monitoring by TLC and spectroscopic validation (IR, NMR) is critical .
Q. How can spectroscopic techniques (IR, NMR, MS) confirm the structure and purity of this compound?
- Methodology :
- IR : Detect O–H stretching (~3200–3550 cm⁻¹) and C–O vibrations (~1050–1250 cm⁻¹) from the methanol group. Thiazole ring C=N and C–S stretches appear at ~1550–1650 cm⁻¹ and ~650–750 cm⁻¹, respectively .
- ¹H NMR : The hydroxyl proton (δ 4.5–5.5 ppm, broad) and thiazole protons (δ 7.5–8.5 ppm) are diagnostic. Coupling constants resolve substituent positions .
- MS : Molecular ion peaks (e.g., m/z 127 for the parent ion) and fragmentation patterns validate structural integrity .
Advanced Research Questions
Q. What strategies resolve hydrogen-bonding interactions of this compound in crystal lattices?
- Methodology : Use single-crystal X-ray diffraction (SHELX programs) to determine H-bond motifs. Graph set analysis (e.g., Etter’s formalism) classifies interactions (e.g., R₂²(8) rings). Validate geometries with software like PLATON to detect outliers in H-bond distances/angles .
Q. How do experimental and computational approaches elucidate the corrosion inhibition mechanism of this compound on metals?
- Methodology :
- Experimental : Electrochemical impedance spectroscopy (EIS) and polarization curves quantify inhibition efficiency in acidic media. Surface analysis (SEM/EDS) confirms adsorption .
- Computational : Density Functional Theory (DFT) calculates Fukui indices to identify nucleophilic/electrophilic sites. Molecular dynamics (MD) simulations model adsorption energies on Fe or steel surfaces .
Q. What methodologies address contradictions in biological activity data of this compound derivatives?
- Methodology : Cross-validate assays (e.g., antimicrobial vs. cytotoxicity) under standardized conditions (pH, temperature). Use structure-activity relationship (SAR) models to isolate substituent effects. Reproducibility requires strict control of synthetic routes (e.g., solvent purity, stoichiometry) .
Q. How do solvent polarity and reaction medium influence regioselectivity in nucleophilic substitutions of this compound?
- Methodology : Polar aprotic solvents (e.g., DMF) stabilize transition states in SN2 reactions at the –CH₂OH group. PEG-400 enhances regioselectivity by reducing side reactions via micellar encapsulation. Kinetic studies (HPLC monitoring) track intermediate formation .
Properties
IUPAC Name |
1,3-thiazol-2-ylmethanol | |
---|---|---|
Source | PubChem | |
URL | https://pubchem.ncbi.nlm.nih.gov | |
Description | Data deposited in or computed by PubChem | |
InChI |
InChI=1S/C4H5NOS/c6-3-4-5-1-2-7-4/h1-2,6H,3H2 | |
Source | PubChem | |
URL | https://pubchem.ncbi.nlm.nih.gov | |
Description | Data deposited in or computed by PubChem | |
InChI Key |
JNHDLNXNYPLBMJ-UHFFFAOYSA-N | |
Source | PubChem | |
URL | https://pubchem.ncbi.nlm.nih.gov | |
Description | Data deposited in or computed by PubChem | |
Canonical SMILES |
C1=CSC(=N1)CO | |
Source | PubChem | |
URL | https://pubchem.ncbi.nlm.nih.gov | |
Description | Data deposited in or computed by PubChem | |
Molecular Formula |
C4H5NOS | |
Source | PubChem | |
URL | https://pubchem.ncbi.nlm.nih.gov | |
Description | Data deposited in or computed by PubChem | |
DSSTOX Substance ID |
DTXSID80383585 | |
Record name | 1,3-Thiazol-2-ylmethanol | |
Source | EPA DSSTox | |
URL | https://comptox.epa.gov/dashboard/DTXSID80383585 | |
Description | DSSTox provides a high quality public chemistry resource for supporting improved predictive toxicology. | |
Molecular Weight |
115.16 g/mol | |
Source | PubChem | |
URL | https://pubchem.ncbi.nlm.nih.gov | |
Description | Data deposited in or computed by PubChem | |
CAS No. |
14542-12-2 | |
Record name | 1,3-Thiazol-2-ylmethanol | |
Source | EPA DSSTox | |
URL | https://comptox.epa.gov/dashboard/DTXSID80383585 | |
Description | DSSTox provides a high quality public chemistry resource for supporting improved predictive toxicology. | |
Record name | 1,3-thiazol-2-ylmethanol | |
Source | European Chemicals Agency (ECHA) | |
URL | https://echa.europa.eu/information-on-chemicals | |
Description | The European Chemicals Agency (ECHA) is an agency of the European Union which is the driving force among regulatory authorities in implementing the EU's groundbreaking chemicals legislation for the benefit of human health and the environment as well as for innovation and competitiveness. | |
Explanation | Use of the information, documents and data from the ECHA website is subject to the terms and conditions of this Legal Notice, and subject to other binding limitations provided for under applicable law, the information, documents and data made available on the ECHA website may be reproduced, distributed and/or used, totally or in part, for non-commercial purposes provided that ECHA is acknowledged as the source: "Source: European Chemicals Agency, http://echa.europa.eu/". Such acknowledgement must be included in each copy of the material. ECHA permits and encourages organisations and individuals to create links to the ECHA website under the following cumulative conditions: Links can only be made to webpages that provide a link to the Legal Notice page. | |
Retrosynthesis Analysis
AI-Powered Synthesis Planning: Our tool employs the Template_relevance Pistachio, Template_relevance Bkms_metabolic, Template_relevance Pistachio_ringbreaker, Template_relevance Reaxys, Template_relevance Reaxys_biocatalysis model, leveraging a vast database of chemical reactions to predict feasible synthetic routes.
One-Step Synthesis Focus: Specifically designed for one-step synthesis, it provides concise and direct routes for your target compounds, streamlining the synthesis process.
Accurate Predictions: Utilizing the extensive PISTACHIO, BKMS_METABOLIC, PISTACHIO_RINGBREAKER, REAXYS, REAXYS_BIOCATALYSIS database, our tool offers high-accuracy predictions, reflecting the latest in chemical research and data.
Strategy Settings
Precursor scoring | Relevance Heuristic |
---|---|
Min. plausibility | 0.01 |
Model | Template_relevance |
Template Set | Pistachio/Bkms_metabolic/Pistachio_ringbreaker/Reaxys/Reaxys_biocatalysis |
Top-N result to add to graph | 6 |
Feasible Synthetic Routes
Disclaimer and Information on In-Vitro Research Products
Please be aware that all articles and product information presented on BenchChem are intended solely for informational purposes. The products available for purchase on BenchChem are specifically designed for in-vitro studies, which are conducted outside of living organisms. In-vitro studies, derived from the Latin term "in glass," involve experiments performed in controlled laboratory settings using cells or tissues. It is important to note that these products are not categorized as medicines or drugs, and they have not received approval from the FDA for the prevention, treatment, or cure of any medical condition, ailment, or disease. We must emphasize that any form of bodily introduction of these products into humans or animals is strictly prohibited by law. It is essential to adhere to these guidelines to ensure compliance with legal and ethical standards in research and experimentation.