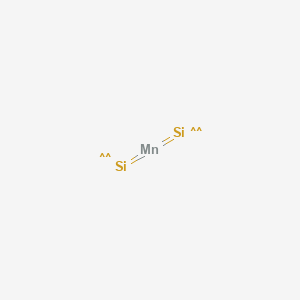
Manganese silicide
Overview
Description
Manganese silicide is a compound composed of manganese and silicon. It is known for its unique properties, including high thermal stability and excellent thermoelectric performance. This compound is often used in various industrial applications, particularly in the field of thermoelectric materials, due to its ability to convert heat into electricity efficiently .
Mechanism of Action
Target of Action
Manganese silicide (MnSi) primarily targets the thermoelectric applications . It is an environmentally friendly p-type thermoelectric material with attractive performance and high stability in the intermediate temperature range (500–800 K) . It is also used as a barrier to diffusion of copper, water, and oxygen in computer chips .
Mode of Action
This compound interacts with its targets through a process known as solid phase reaction (SPR) . In this process, manganese is deposited on a silicon substrate and annealed at different temperatures . This leads to the formation of different manganese silicides . The formation of new Mn17Si30 compound through selective solid-state reaction synthesis has also been reported .
Biochemical Pathways
This compound affects the thermoelectric properties of materials . It is part of the Nowotny chimney ladder (NCL) phases, which are intermetallic binary compounds that typically crystallize in a tetragonal crystal structure . The rich solid-state chemistry of NCL phases inherits fascinating lattice dynamics with unique abilities for structural modifications .
Pharmacokinetics
Itstransport properties are crucial in its applications . For instance, the Hall measurements on the both investigated materials reveal their p-type conductivity and degenerate nature .
Result of Action
The result of this compound’s action is the creation of a material with excellent thermoelectric performance . For example, the Ge doped sample Mn(Ge 0.015 Si 0.985) 1.75 prepared via the TE–PAS technique exhibits a maximum ZT of 0.62 at 840 K . This demonstrates that the TE–PAS technique is a versatile route for rapid fabrication of HMS and other thermoelectric materials .
Action Environment
The action of this compound is influenced by environmental factors such as temperature . For instance, the reaction temperature influences the delivery of Mn vapor to the substrate surface . Also, the thermoelectric performance of this compound is stable in the intermediate temperature range (500–800 K) .
Biochemical Analysis
Biochemical Properties
Manganese silicide plays a crucial role in biochemical reactions, particularly in the context of its interactions with enzymes, proteins, and other biomolecules. It has been observed to interact with various enzymes, including manganese superoxide dismutase (MnSOD), which is essential for protecting cells from oxidative damage by catalyzing the dismutation of superoxide radicals into oxygen and hydrogen peroxide . Additionally, this compound can interact with proteins involved in cellular signaling pathways, influencing their activity and stability.
Cellular Effects
This compound has been shown to affect various types of cells and cellular processes. It influences cell function by modulating cell signaling pathways, gene expression, and cellular metabolism. For instance, this compound can enhance the activity of MnSOD, leading to increased protection against oxidative stress . Furthermore, it has been reported to affect the expression of genes involved in antioxidant defense mechanisms, thereby contributing to cellular homeostasis .
Molecular Mechanism
The molecular mechanism of this compound involves its interaction with biomolecules at the molecular level. This compound can bind to specific sites on enzymes, leading to either inhibition or activation of their catalytic activity . For example, its interaction with MnSOD enhances the enzyme’s ability to neutralize reactive oxygen species, thereby protecting cells from oxidative damage . Additionally, this compound can influence gene expression by modulating transcription factors and other regulatory proteins .
Temporal Effects in Laboratory Settings
In laboratory settings, the effects of this compound have been observed to change over time. Studies have shown that this compound exhibits stability under various conditions, with minimal degradation over extended periods . Long-term exposure to this compound in in vitro and in vivo studies has demonstrated sustained protective effects against oxidative stress and maintenance of cellular function .
Dosage Effects in Animal Models
The effects of this compound vary with different dosages in animal models. At low doses, this compound has been shown to enhance antioxidant defense mechanisms without causing adverse effects . At high doses, it can lead to toxicity and adverse effects, including oxidative damage and disruption of cellular homeostasis . Threshold effects have been observed, indicating that there is an optimal dosage range for achieving beneficial effects without toxicity.
Metabolic Pathways
This compound is involved in various metabolic pathways, interacting with enzymes and cofactors essential for cellular metabolism. It has been shown to influence metabolic flux and metabolite levels by modulating the activity of key enzymes involved in energy production and antioxidant defense . For instance, this compound can enhance the activity of MnSOD, leading to increased production of hydrogen peroxide and subsequent activation of downstream antioxidant pathways .
Transport and Distribution
Within cells and tissues, this compound is transported and distributed through specific transporters and binding proteins. It has been observed to interact with manganese transporters, facilitating its uptake and distribution within cells . Additionally, this compound can bind to proteins involved in intracellular transport, influencing its localization and accumulation in specific cellular compartments .
Subcellular Localization
The subcellular localization of this compound is critical for its activity and function. It has been found to localize in various cellular compartments, including mitochondria, where it exerts its protective effects against oxidative stress . The targeting of this compound to specific organelles is mediated by targeting signals and post-translational modifications that direct it to these compartments . This localization is essential for its role in modulating cellular processes and maintaining cellular homeostasis.
Preparation Methods
Synthetic Routes and Reaction Conditions: Manganese silicide can be synthesized through several methods, including solid-state reactions, chemical vapor deposition, and thermal explosion techniques. One common method involves the solid-state reaction of manganese and silicon powders at high temperatures. The reaction typically occurs at temperatures above 1000°C, resulting in the formation of this compound .
Industrial Production Methods: In industrial settings, this compound is often produced using the thermal explosion method. This process involves the rapid heating of a mixture of manganese and silicon powders, leading to an exothermic reaction that forms this compound. The resulting product is then subjected to plasma-activated sintering to obtain dense bulk samples .
Chemical Reactions Analysis
Types of Reactions: Manganese silicide undergoes various chemical reactions, including oxidation, reduction, and substitution reactions. These reactions are influenced by the specific conditions and reagents used.
Common Reagents and Conditions:
Oxidation: this compound can be oxidized in the presence of oxygen at elevated temperatures, forming manganese oxide and silicon dioxide.
Reduction: Reduction reactions typically involve the use of reducing agents such as hydrogen gas, which can reduce this compound to its elemental components.
Major Products: The major products formed from these reactions include manganese oxide, silicon dioxide, and various substituted silicides, depending on the specific reaction conditions .
Scientific Research Applications
Manganese silicide has a wide range of scientific research applications, including:
Thermoelectric Materials: Due to its excellent thermoelectric properties, this compound is extensively studied for use in thermoelectric generators, which convert waste heat into electricity.
Photovoltaic Devices: this compound is also explored for its potential use in photovoltaic devices, where it can be used to enhance the efficiency of solar cells.
Comparison with Similar Compounds
- Magnesium Silicide
- Iron Silicide
- Cobalt Silicide
- Nickel Silicide
Manganese silicide’s combination of properties makes it a valuable material for a wide range of scientific and industrial applications.
Properties
InChI |
InChI=1S/Mn.2Si | |
---|---|---|
Source | PubChem | |
URL | https://pubchem.ncbi.nlm.nih.gov | |
Description | Data deposited in or computed by PubChem | |
InChI Key |
FHTCLMVMBMJAEE-UHFFFAOYSA-N | |
Source | PubChem | |
URL | https://pubchem.ncbi.nlm.nih.gov | |
Description | Data deposited in or computed by PubChem | |
Canonical SMILES |
[Si]=[Mn]=[Si] | |
Source | PubChem | |
URL | https://pubchem.ncbi.nlm.nih.gov | |
Description | Data deposited in or computed by PubChem | |
Molecular Formula |
MnSi2 | |
Source | PubChem | |
URL | https://pubchem.ncbi.nlm.nih.gov | |
Description | Data deposited in or computed by PubChem | |
Molecular Weight |
111.11 g/mol | |
Source | PubChem | |
URL | https://pubchem.ncbi.nlm.nih.gov | |
Description | Data deposited in or computed by PubChem | |
CAS No. |
12032-86-9 | |
Record name | Manganese silicide (MnSi2) | |
Source | CAS Common Chemistry | |
URL | https://commonchemistry.cas.org/detail?cas_rn=12032-86-9 | |
Description | CAS Common Chemistry is an open community resource for accessing chemical information. Nearly 500,000 chemical substances from CAS REGISTRY cover areas of community interest, including common and frequently regulated chemicals, and those relevant to high school and undergraduate chemistry classes. This chemical information, curated by our expert scientists, is provided in alignment with our mission as a division of the American Chemical Society. | |
Explanation | The data from CAS Common Chemistry is provided under a CC-BY-NC 4.0 license, unless otherwise stated. | |
Record name | Manganese silicide (MnSi2) | |
Source | ChemIDplus | |
URL | https://pubchem.ncbi.nlm.nih.gov/substance/?source=chemidplus&sourceid=0012032869 | |
Description | ChemIDplus is a free, web search system that provides access to the structure and nomenclature authority files used for the identification of chemical substances cited in National Library of Medicine (NLM) databases, including the TOXNET system. | |
Record name | Manganese silicide (MnSi2) | |
Source | EPA Chemicals under the TSCA | |
URL | https://www.epa.gov/chemicals-under-tsca | |
Description | EPA Chemicals under the Toxic Substances Control Act (TSCA) collection contains information on chemicals and their regulations under TSCA, including non-confidential content from the TSCA Chemical Substance Inventory and Chemical Data Reporting. | |
Record name | Manganese disilicide | |
Source | European Chemicals Agency (ECHA) | |
URL | https://echa.europa.eu/substance-information/-/substanceinfo/100.031.606 | |
Description | The European Chemicals Agency (ECHA) is an agency of the European Union which is the driving force among regulatory authorities in implementing the EU's groundbreaking chemicals legislation for the benefit of human health and the environment as well as for innovation and competitiveness. | |
Explanation | Use of the information, documents and data from the ECHA website is subject to the terms and conditions of this Legal Notice, and subject to other binding limitations provided for under applicable law, the information, documents and data made available on the ECHA website may be reproduced, distributed and/or used, totally or in part, for non-commercial purposes provided that ECHA is acknowledged as the source: "Source: European Chemicals Agency, http://echa.europa.eu/". Such acknowledgement must be included in each copy of the material. ECHA permits and encourages organisations and individuals to create links to the ECHA website under the following cumulative conditions: Links can only be made to webpages that provide a link to the Legal Notice page. | |
Q1: What is the typical stoichiometry of higher manganese silicides (HMS)?
A1: HMS typically exhibit an irrational stoichiometry, often represented as MnSiγ, where γ falls between 1.73 and 1.75. This "odd stoichiometry" is a defining characteristic of their unique crystal structure. []
Q2: What is the crystal structure of higher manganese silicides?
A2: HMS crystallize in a complex structure known as the Nowotny Chimney Ladder (NCL) phase. This structure is characterized by a tetragonal unit cell with a large number of atoms. [, , ] The complexity of this structure contributes to their interesting thermoelectric properties.
Q3: Are there different phases of higher manganese silicides?
A3: Yes, several distinct phases exist within the HMS family, including Mn11Si19, Mn15Si26, Mn27Si47, and Mn4Si7. These phases differ slightly in their Mn/Si ratio, leading to variations in their electronic band structures and thermoelectric properties. []
Q4: What is the significance of the "odd stoichiometry" in HMS?
A4: The odd stoichiometry of HMS is directly related to the formation of their characteristic NCL structure. This structure, with its inherent anharmonicity, plays a crucial role in the material's low thermal conductivity, a key factor in its thermoelectric efficiency. []
Q5: How does the presence of secondary phases affect HMS properties?
A5: Secondary phases, such as MnSi and Si, are often observed in HMS, especially those synthesized via traditional melting techniques. These phases can significantly impact the thermoelectric properties, often leading to a decrease in the Seebeck coefficient and an increase in electrical and thermal conductivity. [, , ]
Q6: Can manganese silicides exist in nanostructured forms?
A6: Yes, manganese silicides have been successfully synthesized in various nanostructured forms, including nanowires and nanoribbons. [, , ] These nanostructures offer the potential for enhanced thermoelectric performance due to increased phonon scattering at grain boundaries.
Q7: What are the common methods for synthesizing higher manganese silicides?
A7: HMS are typically synthesized using solid-state reactions, which involve mixing and heating elemental manganese and silicon powders at high temperatures. Other methods include chemical vapor deposition (CVD) [, ], melt spinning [, ], and reactive deposition epitaxy [].
Q8: How does the synthesis method influence the properties of HMS?
A8: The synthesis method can significantly influence the microstructure and phase purity of HMS, ultimately affecting their thermoelectric properties. For example, melt spinning, a rapid solidification technique, can produce nanostructured HMS with enhanced thermoelectric performance compared to traditional solid-state synthesis. []
Q9: What is the role of doping in tailoring HMS properties?
A9: Doping HMS with elements like aluminum, germanium, and chromium can effectively tune their carrier concentration, influencing their electrical conductivity and Seebeck coefficient. [, , ] This enables optimization of their thermoelectric properties for specific temperature ranges.
Q10: What are the challenges associated with achieving high-quality HMS films?
A10: Fabricating high-quality HMS films presents several challenges, including controlling the stoichiometry, minimizing interfacial reactions with the substrate, and achieving uniform film thickness. [, , , ]
Q11: Why are manganese silicides considered promising for thermoelectric applications?
A11: HMS are attractive for thermoelectric applications due to their favorable combination of properties, including a high Seebeck coefficient, low thermal conductivity, abundance of constituent elements, and good thermal stability at elevated temperatures. [, , ]
Q12: What are the potential applications of HMS in thermoelectric devices?
A12: HMS are particularly well-suited for waste heat recovery applications in the medium temperature range (500–700 °C). Potential applications include converting waste heat from industrial processes, automotive exhaust, and solar thermal systems into usable electricity. [, ]
Q13: How can the thermoelectric performance of HMS be further improved?
A13: Ongoing research focuses on enhancing the ZT of HMS through various strategies, including nanostructuring to reduce thermal conductivity [, , ], doping to optimize carrier concentration [, , ], and exploring new compositions and synthesis techniques.
Disclaimer and Information on In-Vitro Research Products
Please be aware that all articles and product information presented on BenchChem are intended solely for informational purposes. The products available for purchase on BenchChem are specifically designed for in-vitro studies, which are conducted outside of living organisms. In-vitro studies, derived from the Latin term "in glass," involve experiments performed in controlled laboratory settings using cells or tissues. It is important to note that these products are not categorized as medicines or drugs, and they have not received approval from the FDA for the prevention, treatment, or cure of any medical condition, ailment, or disease. We must emphasize that any form of bodily introduction of these products into humans or animals is strictly prohibited by law. It is essential to adhere to these guidelines to ensure compliance with legal and ethical standards in research and experimentation.