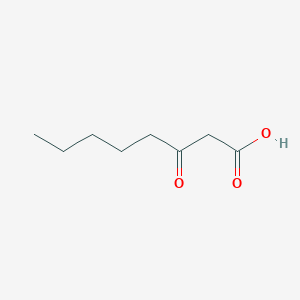
3-Oxooctanoic acid
Overview
Description
3-Oxooctanoic acid, also known as beta-oxocaprylic acid, is a derivative of octanoic acid carrying a beta-oxo group. It is a medium-chain keto acid with the molecular formula C8H14O3. This compound is a possible metabolite of 2-octynoic acid and has been detected in various organisms, including yeast, plants, and humans .
Mechanism of Action
Target of Action
3-Oxooctanoic acid is a primary metabolite . Primary metabolites are metabolically or physiologically essential metabolites that are directly involved in an organism’s growth, development, or reproduction . It is a derivative of octanoic acid carrying a β-oxo group .
Mode of Action
As a β-oxo fatty acid , it may participate in various metabolic processes, including the synthesis, decomposition, and metabolism of fatty acids .
Biochemical Pathways
This compound is a possible metabolite of 2-octynoic acid . It is involved in the metabolism of fatty acids, where it can be converted into acetyl-CoA through beta oxidation, and then enter the citric acid cycle .
Result of Action
As a primary metabolite, it is likely to play a role in various physiological processes, including growth, development, and reproduction .
Biochemical Analysis
Biochemical Properties
3-Oxooctanoic acid is a potential metabolite of 2-octynoic acid . It is a Bronsted acid, a molecular entity capable of donating a hydron to an acceptor
Cellular Effects
As a primary metabolite, it is likely to be involved in essential metabolic or physiological processes .
Molecular Mechanism
As a potential metabolite of 2-octynoic acid, it may be involved in the metabolism of this compound .
Metabolic Pathways
Preparation Methods
Synthetic Routes and Reaction Conditions: 3-Oxooctanoic acid can be synthesized through the enzymatic hydration of 2-octynoic acid. This process involves the addition of water to the triple bond of 2-octynoic acid, resulting in the formation of this compound .
Industrial Production Methods: This method typically requires controlled reaction conditions to ensure the selective formation of the beta-oxo group .
Chemical Reactions Analysis
Types of Reactions: 3-Oxooctanoic acid undergoes various chemical reactions, including:
Oxidation: The compound can be further oxidized to form dicarboxylic acids.
Reduction: Reduction of the keto group can yield the corresponding alcohol.
Substitution: The beta-oxo group can participate in nucleophilic substitution reactions.
Common Reagents and Conditions:
Oxidation: Common oxidizing agents include potassium permanganate and chromium trioxide.
Reduction: Reducing agents such as sodium borohydride or lithium aluminum hydride are used.
Substitution: Nucleophiles like amines or alcohols can react with the beta-oxo group under acidic or basic conditions.
Major Products Formed:
Oxidation: Formation of medium-chain dicarboxylic acids.
Reduction: Formation of 3-hydroxyoctanoic acid.
Substitution: Formation of various substituted derivatives depending on the nucleophile used.
Scientific Research Applications
3-Oxooctanoic acid has several scientific research applications:
Chemistry: Used as an intermediate in organic synthesis and as a building block for more complex molecules.
Biology: Studied as a metabolite in various organisms, providing insights into metabolic pathways.
Medicine: Potential biomarker for certain metabolic disorders and diseases.
Industry: Utilized in the production of specialty chemicals and materials
Comparison with Similar Compounds
3-Hydroxyoctanoic acid: A reduced form of 3-oxooctanoic acid with a hydroxyl group instead of a keto group.
Octanoic acid: The parent compound without the beta-oxo group.
2-Octynoic acid: A precursor to this compound through enzymatic hydration
Uniqueness: this compound is unique due to its beta-oxo group, which imparts distinct chemical reactivity and metabolic roles compared to its similar compounds. This functional group allows it to participate in specific oxidation, reduction, and substitution reactions, making it valuable in various scientific and industrial applications .
Properties
IUPAC Name |
3-oxooctanoic acid | |
---|---|---|
Source | PubChem | |
URL | https://pubchem.ncbi.nlm.nih.gov | |
Description | Data deposited in or computed by PubChem | |
InChI |
InChI=1S/C8H14O3/c1-2-3-4-5-7(9)6-8(10)11/h2-6H2,1H3,(H,10,11) | |
Source | PubChem | |
URL | https://pubchem.ncbi.nlm.nih.gov | |
Description | Data deposited in or computed by PubChem | |
InChI Key |
FWNRRWJFOZIGQZ-UHFFFAOYSA-N | |
Source | PubChem | |
URL | https://pubchem.ncbi.nlm.nih.gov | |
Description | Data deposited in or computed by PubChem | |
Canonical SMILES |
CCCCCC(=O)CC(=O)O | |
Source | PubChem | |
URL | https://pubchem.ncbi.nlm.nih.gov | |
Description | Data deposited in or computed by PubChem | |
Molecular Formula |
C8H14O3 | |
Source | PubChem | |
URL | https://pubchem.ncbi.nlm.nih.gov | |
Description | Data deposited in or computed by PubChem | |
DSSTOX Substance ID |
DTXSID10157812 | |
Record name | 3-Ketooctanoic acid | |
Source | EPA DSSTox | |
URL | https://comptox.epa.gov/dashboard/DTXSID10157812 | |
Description | DSSTox provides a high quality public chemistry resource for supporting improved predictive toxicology. | |
Molecular Weight |
158.19 g/mol | |
Source | PubChem | |
URL | https://pubchem.ncbi.nlm.nih.gov | |
Description | Data deposited in or computed by PubChem | |
Physical Description |
Solid | |
Record name | 3-Oxooctanoic acid | |
Source | Human Metabolome Database (HMDB) | |
URL | http://www.hmdb.ca/metabolites/HMDB0010721 | |
Description | The Human Metabolome Database (HMDB) is a freely available electronic database containing detailed information about small molecule metabolites found in the human body. | |
Explanation | HMDB is offered to the public as a freely available resource. Use and re-distribution of the data, in whole or in part, for commercial purposes requires explicit permission of the authors and explicit acknowledgment of the source material (HMDB) and the original publication (see the HMDB citing page). We ask that users who download significant portions of the database cite the HMDB paper in any resulting publications. | |
CAS No. |
13283-91-5 | |
Record name | 3-Oxooctanoic acid | |
Source | CAS Common Chemistry | |
URL | https://commonchemistry.cas.org/detail?cas_rn=13283-91-5 | |
Description | CAS Common Chemistry is an open community resource for accessing chemical information. Nearly 500,000 chemical substances from CAS REGISTRY cover areas of community interest, including common and frequently regulated chemicals, and those relevant to high school and undergraduate chemistry classes. This chemical information, curated by our expert scientists, is provided in alignment with our mission as a division of the American Chemical Society. | |
Explanation | The data from CAS Common Chemistry is provided under a CC-BY-NC 4.0 license, unless otherwise stated. | |
Record name | 3-Ketooctanoic acid | |
Source | ChemIDplus | |
URL | https://pubchem.ncbi.nlm.nih.gov/substance/?source=chemidplus&sourceid=0013283915 | |
Description | ChemIDplus is a free, web search system that provides access to the structure and nomenclature authority files used for the identification of chemical substances cited in National Library of Medicine (NLM) databases, including the TOXNET system. | |
Record name | 3-Ketooctanoic acid | |
Source | EPA DSSTox | |
URL | https://comptox.epa.gov/dashboard/DTXSID10157812 | |
Description | DSSTox provides a high quality public chemistry resource for supporting improved predictive toxicology. | |
Synthesis routes and methods
Procedure details
Retrosynthesis Analysis
AI-Powered Synthesis Planning: Our tool employs the Template_relevance Pistachio, Template_relevance Bkms_metabolic, Template_relevance Pistachio_ringbreaker, Template_relevance Reaxys, Template_relevance Reaxys_biocatalysis model, leveraging a vast database of chemical reactions to predict feasible synthetic routes.
One-Step Synthesis Focus: Specifically designed for one-step synthesis, it provides concise and direct routes for your target compounds, streamlining the synthesis process.
Accurate Predictions: Utilizing the extensive PISTACHIO, BKMS_METABOLIC, PISTACHIO_RINGBREAKER, REAXYS, REAXYS_BIOCATALYSIS database, our tool offers high-accuracy predictions, reflecting the latest in chemical research and data.
Strategy Settings
Precursor scoring | Relevance Heuristic |
---|---|
Min. plausibility | 0.01 |
Model | Template_relevance |
Template Set | Pistachio/Bkms_metabolic/Pistachio_ringbreaker/Reaxys/Reaxys_biocatalysis |
Top-N result to add to graph | 6 |
Feasible Synthetic Routes
Q1: What is the significance of 3-oxooctanoic acid in natural product synthesis?
A1: this compound serves as a valuable building block in the synthesis of various natural products. For instance, it is a key precursor in the synthesis of syringolide 1, a bioactive compound found in bacteria. Researchers have successfully synthesized both (−)- and (+)-Syringolide 1 from 2,3-O-isopropylidene-β-threo-pentulofuranose, utilizing this compound as a starting material []. This highlights the compound's versatility in constructing complex molecular structures found in nature.
Q2: Can this compound be used to produce halogenated marine natural products?
A2: Yes, this compound plays a crucial role in the biosynthesis of halogenated marine natural products. Studies have shown that bromoperoxidase enzymes, found in marine algae like Penicillus capitatus, can catalyze the bromination of this compound []. This enzymatic reaction leads to the formation of various brominated compounds, including 1-bromo-2-heptanone, 1,1-dibromo-2-heptanone, 1,1,1-tribromo-2-heptanone, and bromoform []. These findings suggest a potential biosynthetic route for halogenated hydrocarbons found in marine environments.
Q3: How can this compound be asymmetrically reduced?
A3: Fermenting baker's yeast offers an effective method for the asymmetric reduction of this compound to its corresponding (R)-hydroxy acid []. This biocatalytic approach provides high enantioselectivity (≥98% ee) for a range of β-keto acids, including this compound, highlighting its potential for producing chiral building blocks for various applications [].
Q4: Are there any novel peptide-polyketide compounds derived from this compound?
A4: Yes, a recent discovery revealed a novel peptide-polyketide compound named dahurelmusin A, isolated from Elymus dahuricus infected with the Epichloë bromicola endophyte []. Dahurelmusin A features an unprecedented 5-hydroxy-2,2,4,6-tetramethyl-3-oxooctanoic acid moiety, showcasing the structural diversity possible within this class of compounds []. Interestingly, dahurelmusin A also exhibits insecticidal activities against aphids and cabbage aphids [], suggesting potential applications in pest control.
Q5: What are the analytical techniques used to study this compound and its derivatives?
A5: Researchers employ a combination of analytical techniques to characterize and quantify this compound and its derivatives. Gas chromatography coupled with mass spectrometry (GC-MS) has been instrumental in identifying brominated products derived from this compound in enzymatic reactions []. Additionally, spectroscopic methods like nuclear magnetic resonance (NMR) and X-ray crystallography are crucial for structural elucidation and stereochemical determination of novel compounds like dahurelmusin A [].
Disclaimer and Information on In-Vitro Research Products
Please be aware that all articles and product information presented on BenchChem are intended solely for informational purposes. The products available for purchase on BenchChem are specifically designed for in-vitro studies, which are conducted outside of living organisms. In-vitro studies, derived from the Latin term "in glass," involve experiments performed in controlled laboratory settings using cells or tissues. It is important to note that these products are not categorized as medicines or drugs, and they have not received approval from the FDA for the prevention, treatment, or cure of any medical condition, ailment, or disease. We must emphasize that any form of bodily introduction of these products into humans or animals is strictly prohibited by law. It is essential to adhere to these guidelines to ensure compliance with legal and ethical standards in research and experimentation.