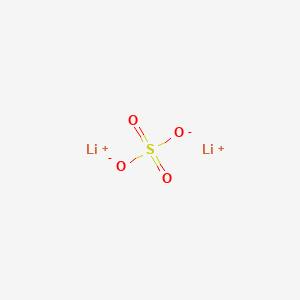
Lithium sulfate
Overview
Description
Lithium sulfate is an inorganic compound with the chemical formula Li₂SO₄. It is the lithium salt of sulfuric acid and appears as a white crystalline solid. This compound is known for its hygroscopic nature, meaning it can absorb moisture from the air.
Mechanism of Action
Target of Action
Lithium sulfate is an inorganic salt and the lithium salt of sulfuric acid .
Mode of Action
One proposed mechanism is the non-competitive inhibition of an enzyme called inositol monophosphatase . Another theory suggests that lithium’s action may be enhanced through the deactivation of the GSK-3B enzyme . The regulation of GSK-3B by lithium may affect the circadian clock .
Biochemical Pathways
This compound may affect several biochemical pathways. For instance, it’s suggested that IP3 binds to specific receptors to help open the calcium (Ca 2+) channel, and DAG initiates activation of protein kinase C (PKC) . .
Pharmacokinetics
The pharmacokinetics of lithium is linear within the dose regimen used in clinical practice . Lithium has an extremely narrow therapeutic window, and therapeutic drug monitoring (TDM) is necessary to avoid adverse effects or lack of drug response . Several factors can affect lithium pharmacokinetics, such as age, body weight, renal function, and drug-drug interactions .
Result of Action
Lithium has been shown to have many widely varying biochemical and phenomenological effects, suggesting that a systems biology approach is required to understand its action . Multiple lines of evidence point to lithium as a significant factor in the development of cancer .
Action Environment
Environmental factors can influence the action, efficacy, and stability of this compound. For instance, the solubility of this compound in water decreases with increasing temperature, as its dissolution is an exothermic process . This relatively unusual property, also called retrograde solubility, is shared with few inorganic compounds . Furthermore, leaching of lithium from discharged batteries, as well as its subsequent migration through soil and water, represents serious environmental hazards .
Biochemical Analysis
. .
Biochemical Properties
It is known to be crucial in the preparation of crystal structures for biochemical assays . This facilitates the deciphering of complex biological structures .
Temporal Effects in Laboratory Settings
In laboratory settings, lithium sulfate is used in the preparation of crystal structures for biochemical assays
Preparation Methods
Synthetic Routes and Reaction Conditions: Lithium sulfate can be synthesized through several methods. One common laboratory method involves the reaction of lithium hydroxide (LiOH) or lithium carbonate (Li₂CO₃) with sulfuric acid (H₂SO₄): [ \text{LiOH} + \text{H₂SO₄} \rightarrow \text{Li₂SO₄} + \text{H₂O} ] [ \text{Li₂CO₃} + \text{H₂SO₄} \rightarrow \text{Li₂SO₄} + \text{H₂O} + \text{CO₂} ]
Industrial Production Methods: Industrially, this compound is often produced as a byproduct in the extraction of lithium from spodumene (a lithium-rich mineral) or from lithium brine sources. The process involves roasting the mineral with sulfuric acid, followed by leaching and purification steps to isolate this compound .
Types of Reactions:
-
Oxidation and Reduction: this compound can undergo reduction reactions to form lithium sulfide (Li₂S) when reacted with hydrogen gas (H₂) at high temperatures. [ \text{Li₂SO₄} + 4\text{H₂} \rightarrow \text{Li₂S} + 4\text{H₂O} ]
-
Substitution Reactions: this compound can participate in metathesis reactions with other salts. For example, reacting this compound with sodium sulfide (Na₂S) can produce lithium sulfide and sodium sulfate (Na₂SO₄). [ \text{Li₂SO₄} + \text{Na₂S} \rightarrow \text{Li₂S} + \text{Na₂SO₄} ]
Common Reagents and Conditions:
Hydrogen Gas: Used in reduction reactions to produce lithium sulfide.
Sodium Sulfide: Used in metathesis reactions to produce lithium sulfide.
Major Products:
Scientific Research Applications
Lithium sulfate has a wide range of applications in scientific research:
Biology and Medicine: Lithium salts, including this compound, are studied for their potential neuroprotective effects and their use in treating bipolar disorder.
Industry: this compound is used in the production of specialty glasses and ceramics.
Comparison with Similar Compounds
Lithium Chloride (LiCl): Another lithium salt with similar applications in medicine and industry.
Sodium Sulfate (Na₂SO₄): A sulfate salt with similar chemical properties but different applications.
Potassium Sulfate (K₂SO₄): Another sulfate salt used primarily in fertilizers.
Uniqueness of Lithium Sulfate: this compound is unique due to its retrograde solubility, which is uncommon among salts. This property makes it useful in specific industrial processes where temperature-dependent solubility is advantageous. Additionally, its role in the synthesis of lithium sulfide for advanced battery technologies highlights its importance in the field of energy storage .
Properties
IUPAC Name |
dilithium;sulfate | |
---|---|---|
Source | PubChem | |
URL | https://pubchem.ncbi.nlm.nih.gov | |
Description | Data deposited in or computed by PubChem | |
InChI |
InChI=1S/2Li.H2O4S/c;;1-5(2,3)4/h;;(H2,1,2,3,4)/q2*+1;/p-2 | |
Source | PubChem | |
URL | https://pubchem.ncbi.nlm.nih.gov | |
Description | Data deposited in or computed by PubChem | |
InChI Key |
INHCSSUBVCNVSK-UHFFFAOYSA-L | |
Source | PubChem | |
URL | https://pubchem.ncbi.nlm.nih.gov | |
Description | Data deposited in or computed by PubChem | |
Canonical SMILES |
[Li+].[Li+].[O-]S(=O)(=O)[O-] | |
Source | PubChem | |
URL | https://pubchem.ncbi.nlm.nih.gov | |
Description | Data deposited in or computed by PubChem | |
Molecular Formula |
Li2SO4, Li2O4S | |
Record name | lithium sulfate | |
Source | Wikipedia | |
URL | https://en.wikipedia.org/wiki/Lithium_sulfate | |
Description | Chemical information link to Wikipedia. | |
Source | PubChem | |
URL | https://pubchem.ncbi.nlm.nih.gov | |
Description | Data deposited in or computed by PubChem | |
DSSTOX Substance ID |
DTXSID3049201 | |
Record name | Lithium sulfate | |
Source | EPA DSSTox | |
URL | https://comptox.epa.gov/dashboard/DTXSID3049201 | |
Description | DSSTox provides a high quality public chemistry resource for supporting improved predictive toxicology. | |
Molecular Weight |
110.0 g/mol | |
Source | PubChem | |
URL | https://pubchem.ncbi.nlm.nih.gov | |
Description | Data deposited in or computed by PubChem | |
CAS No. |
10377-48-7 | |
Record name | Lithium sulfate | |
Source | ChemIDplus | |
URL | https://pubchem.ncbi.nlm.nih.gov/substance/?source=chemidplus&sourceid=0010377487 | |
Description | ChemIDplus is a free, web search system that provides access to the structure and nomenclature authority files used for the identification of chemical substances cited in National Library of Medicine (NLM) databases, including the TOXNET system. | |
Record name | Sulfuric acid, lithium salt (1:2) | |
Source | EPA Chemicals under the TSCA | |
URL | https://www.epa.gov/chemicals-under-tsca | |
Description | EPA Chemicals under the Toxic Substances Control Act (TSCA) collection contains information on chemicals and their regulations under TSCA, including non-confidential content from the TSCA Chemical Substance Inventory and Chemical Data Reporting. | |
Record name | Lithium sulfate | |
Source | EPA DSSTox | |
URL | https://comptox.epa.gov/dashboard/DTXSID3049201 | |
Description | DSSTox provides a high quality public chemistry resource for supporting improved predictive toxicology. | |
Record name | Lithium sulphate | |
Source | European Chemicals Agency (ECHA) | |
URL | https://echa.europa.eu/substance-information/-/substanceinfo/100.030.734 | |
Description | The European Chemicals Agency (ECHA) is an agency of the European Union which is the driving force among regulatory authorities in implementing the EU's groundbreaking chemicals legislation for the benefit of human health and the environment as well as for innovation and competitiveness. | |
Explanation | Use of the information, documents and data from the ECHA website is subject to the terms and conditions of this Legal Notice, and subject to other binding limitations provided for under applicable law, the information, documents and data made available on the ECHA website may be reproduced, distributed and/or used, totally or in part, for non-commercial purposes provided that ECHA is acknowledged as the source: "Source: European Chemicals Agency, http://echa.europa.eu/". Such acknowledgement must be included in each copy of the material. ECHA permits and encourages organisations and individuals to create links to the ECHA website under the following cumulative conditions: Links can only be made to webpages that provide a link to the Legal Notice page. | |
Record name | LITHIUM SULFATE | |
Source | FDA Global Substance Registration System (GSRS) | |
URL | https://gsrs.ncats.nih.gov/ginas/app/beta/substances/919XA137JK | |
Description | The FDA Global Substance Registration System (GSRS) enables the efficient and accurate exchange of information on what substances are in regulated products. Instead of relying on names, which vary across regulatory domains, countries, and regions, the GSRS knowledge base makes it possible for substances to be defined by standardized, scientific descriptions. | |
Explanation | Unless otherwise noted, the contents of the FDA website (www.fda.gov), both text and graphics, are not copyrighted. They are in the public domain and may be republished, reprinted and otherwise used freely by anyone without the need to obtain permission from FDA. Credit to the U.S. Food and Drug Administration as the source is appreciated but not required. | |
Retrosynthesis Analysis
AI-Powered Synthesis Planning: Our tool employs the Template_relevance Pistachio, Template_relevance Bkms_metabolic, Template_relevance Pistachio_ringbreaker, Template_relevance Reaxys, Template_relevance Reaxys_biocatalysis model, leveraging a vast database of chemical reactions to predict feasible synthetic routes.
One-Step Synthesis Focus: Specifically designed for one-step synthesis, it provides concise and direct routes for your target compounds, streamlining the synthesis process.
Accurate Predictions: Utilizing the extensive PISTACHIO, BKMS_METABOLIC, PISTACHIO_RINGBREAKER, REAXYS, REAXYS_BIOCATALYSIS database, our tool offers high-accuracy predictions, reflecting the latest in chemical research and data.
Strategy Settings
Precursor scoring | Relevance Heuristic |
---|---|
Min. plausibility | 0.01 |
Model | Template_relevance |
Template Set | Pistachio/Bkms_metabolic/Pistachio_ringbreaker/Reaxys/Reaxys_biocatalysis |
Top-N result to add to graph | 6 |
Feasible Synthetic Routes
Q1: What is the molecular formula and weight of lithium sulfate?
A1: this compound has the molecular formula Li2SO4 and a molecular weight of 109.94 g/mol. []
Q2: Are there any spectroscopic techniques used to characterize this compound?
A2: Yes, X-ray diffraction (XRD) is commonly used to study the crystal structure of this compound, including its changes with temperature. [] Additionally, Fourier-transform infrared spectroscopy (FTIR) can be employed to identify the functional groups present in this compound crystals. []
Q3: What is the thermal behavior of this compound?
A3: this compound exhibits a solid-solid phase transition at 572 °C and melts at 860 °C. [] The heat of fusion for Li2SO4 is 1.76 kcal/mol, and the heat of the solid-solid transition is 5.98 kcal/mol. []
Q4: How does the density of this compound compare to magnesium sulfate?
A4: this compound has a higher density than magnesium sulfate. This difference in density can be utilized to separate the two salts using dense medium separation techniques. []
Q5: How is this compound used in the production of lithium carbonate?
A5: this compound solution serves as a precursor in the production of lithium carbonate (Li2CO3), a key material for lithium-ion batteries. The this compound solution undergoes a carbonization and precipitation reaction at high temperatures to yield high-purity battery-grade lithium carbonate. []
Q6: Can this compound be directly converted into lithium hydroxide?
A6: Yes, this compound can be converted into lithium hydroxide (LiOH) using bipolar membrane electrodialysis. This process simultaneously generates sulfuric acid as a byproduct. []
Q7: What role does this compound play in the extraction of lithium from spodumene?
A7: In some extraction methods, spodumene is reacted with fluoride and sulfuric acid, leading to the formation of this compound in the solid phase. This this compound is then leached out using water. [, ]
Q8: Are there alternative lithium salts used in extraction processes?
A8: Yes, depending on the extraction method, other lithium salts like lithium chloride (LiCl) can also be produced from lithium-containing materials. []
Q9: Can waste materials be utilized for this compound production?
A9: Yes, this compound can be recovered from waste lithium-ion batteries (LIBs) and purified for further use. [] This process contributes to resource recycling and reduces the environmental impact of battery waste. [, ]
Q10: How does this compound impact the performance of lead-acid batteries?
A10: Adding this compound to the electrolyte of lead-acid batteries has been shown to improve both the cycle life and efficiency of the battery compared to using dilute sulfuric acid alone. []
Q11: What is the solubility behavior of this compound in water?
A11: this compound is soluble in water, and its solubility is influenced by factors such as temperature and the presence of other salts. [, ] For instance, the solubility of this compound in water decreases with increasing concentrations of sodium sulfate. []
Q12: Can organic solvents be used to separate lithium salts from solutions?
A12: Yes, amines like triethylamine and diisopropylamine have been investigated for the extractive crystallization of this compound from aqueous solutions. These amines preferentially extract water at low temperatures, leading to the precipitation of this compound crystals. []
Q13: Does this compound form double salts with other alkali metal sulfates?
A13: Yes, this compound readily forms double salts with sodium sulfate and potassium sulfate. In the quaternary system Li2SO4 + Na2SO4 + K2SO4 + H2O, the double salts 3Na2SO4·Li2SO4·12H2O and KLiSO4 are formed. []
Q14: How does temperature affect the phase behavior of aqueous this compound systems?
A14: In aqueous two-phase systems (ATPS) containing polyethylene glycol (PEG) and this compound, increasing the temperature generally leads to an increase in the slope of the tie line and a decrease in the amount of salt required for phase separation. []
Q15: What is the impact of this compound on the properties of cement?
A15: Research shows that this compound, when used in combination with nano silicon carbide and polyvinyl alcohol, can significantly improve the early strength of cement. The lithium ions in this compound react with the calcium hydroxide (Ca(OH)2) produced during cement hydration, promoting the formation of hydration products and enhancing cement strength. []
Q16: Can this compound be used as an electrolyte in electrochemical devices?
A16: Yes, molten this compound, particularly the eutectic mixture with potassium sulfate, exhibits good ionic conductivity at elevated temperatures and can function as an electrolyte in high-temperature electrochemical devices. [, ]
Q17: How does doping affect the ionic conductivity of this compound?
A17: Doping this compound with small amounts of lithium chloride (LiCl) has been observed to increase its ionic conductivity, suggesting a change in the lithium ion transport mechanism. []
Q18: What is the significance of the "AK-factor" in lithium ion diffusion in Li2SO4?
A18: Isotope effect studies suggest that the AK-factor for lithium ion migration in fcc Li2SO4 is close to unity. This indicates that the diffusing lithium ions do not significantly share energy with the anion lattice during their movement. []
Disclaimer and Information on In-Vitro Research Products
Please be aware that all articles and product information presented on BenchChem are intended solely for informational purposes. The products available for purchase on BenchChem are specifically designed for in-vitro studies, which are conducted outside of living organisms. In-vitro studies, derived from the Latin term "in glass," involve experiments performed in controlled laboratory settings using cells or tissues. It is important to note that these products are not categorized as medicines or drugs, and they have not received approval from the FDA for the prevention, treatment, or cure of any medical condition, ailment, or disease. We must emphasize that any form of bodily introduction of these products into humans or animals is strictly prohibited by law. It is essential to adhere to these guidelines to ensure compliance with legal and ethical standards in research and experimentation.