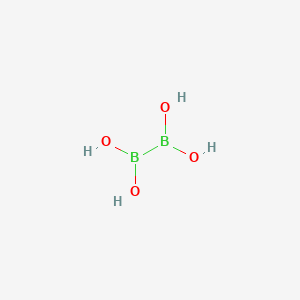
Tetrahydroxydiboron
Overview
Description
Mechanism of Action
Target of Action
Tetrahydroxydiboron, also known as Bis-boric Acid or THDB, is a versatile reagent used in various chemical reactions . Its primary targets include a wide variety of aryl and heteroaryl substrates . It is used in borylation reactions, hydrogenation, catalysis, radical reactions, and H2 generation .
Mode of Action
This compound interacts with its targets through several mechanisms. It serves as a borylation agent, introducing boron atoms into organic molecules . It also acts as a reductant, including in transfer hydrogenation reactions . In the presence of a palladium catalyst, it can transfer hydrogen atoms from water across unsaturated carbon-carbon bonds . Furthermore, it can generate radicals and produce H2 from water upon hydrolysis .
Biochemical Pathways
The biochemical pathways affected by this compound are primarily related to its role as a borylation agent and a source of hydrogen. Borylation reactions are crucial in organic chemistry, particularly in the synthesis of boronic acids . The hydrogen generated from this compound can be used in various reduction reactions .
Pharmacokinetics
Information on the ADME (Absorption, Distribution, Metabolism, and Excretion) properties of this compound is limited. It is known to be a solid substance that is very soluble in water . This solubility suggests that it could be readily absorbed and distributed in an aqueous environment.
Result of Action
The molecular and cellular effects of this compound’s action depend on the specific reaction it is involved in. For instance, in borylation reactions, it introduces boron atoms into organic molecules, altering their structure and properties . When used as a reductant, it can reduce other compounds, changing their oxidation state .
Action Environment
Environmental factors can influence the action of this compound. For example, its ability to generate hydrogen from water suggests that the presence and quality of water could impact its efficacy . Additionally, the temperature can affect its stability and reactivity. When heated to over 90 °C, this compound dehydrates to a polymeric boron (II) oxide .
Biochemical Analysis
Biochemical Properties
Tetrahydroxydiboron is involved in borylation, hydrogenation, catalysis, and radical reactions . It is also known to generate hydrogen from water upon hydrolysis . It interacts with various enzymes and proteins, facilitating a range of biochemical reactions .
Cellular Effects
The cellular effects of this compound are primarily observed in its role as a catalyst and reductant . It influences cell function by participating in various biochemical reactions, potentially impacting cell signaling pathways, gene expression, and cellular metabolism .
Molecular Mechanism
At the molecular level, this compound exerts its effects through binding interactions with biomolecules, enzyme inhibition or activation, and changes in gene expression . It is also involved in transfer hydrogenation reactions to various substrates .
Temporal Effects in Laboratory Settings
In laboratory settings, the effects of this compound can change over time. For instance, when heated to over 90 °C, it dehydrates to a polymeric boron (II) oxide . It also slowly gives off hydrogen gas in a water solution .
Metabolic Pathways
This compound is involved in several metabolic pathways. It interacts with enzymes and cofactors, potentially affecting metabolic flux or metabolite levels .
Preparation Methods
The synthesis of tetrahydroxydiboron involves several steps:
Reaction of Boron Trichloride with Alcohols: Initially reported in 1931, this reaction produces dimethoxyboron chloride (B(OCH₃)₂Cl).
Reduction with Sodium: Egon Wiberg and Wilhelm Ruschmann introduced the boron-boron bond by reducing dimethoxyboron chloride with sodium, forming tetramethoxydiboron (B₂(OCH₃)₄).
Hydrolysis: The tetramethoxydiboron is then hydrolyzed to produce this compound.
The overall reaction can be summarized as: [ 2 BCl₃ + 2 Na + 4 H₂O \rightarrow B₂(OH)₄ + 2 NaCl + 4 HCl ]
Chemical Reactions Analysis
Tetrahydroxydiboron undergoes various chemical reactions:
Dehydration: When heated above 90°C, it dehydrates to form polymeric boron(II) oxide.
Reduction: It acts as a reducing agent, slowly releasing hydrogen gas in aqueous solutions.
Scientific Research Applications
Tetrahydroxydiboron has a wide range of applications in scientific research:
Borylation: It is an efficient borylating agent for a variety of aryl and heteroaryl substrates.
Hydrogenation: It is used in transfer hydrogenation reactions.
Catalysis: It serves as a catalyst in various organic reactions.
Radical Reactions: It generates radicals for use in organic synthesis.
Hydrogen Generation: It produces hydrogen gas upon hydrolysis, which can be used in energy-related applications.
Material Science: It acts as an electron dopant for single-walled carbon nanotubes, enhancing their electrical and optical properties.
Comparison with Similar Compounds
Tetrahydroxydiboron is compared with other diboron compounds such as:
Bis(pinacolato)diboron (B₂pin₂): Widely used in borylation reactions but less reactive due to electronic delocalization.
Diborane (B₂H₆): A simpler boron hydride used in hydroboration reactions.
Diboron tetrafluoride (B₂F₄): Used in various organic transformations.
This compound is unique due to its versatility in borylation, reduction, and hydrogen generation, making it a valuable reagent in both academic and industrial research .
Properties
IUPAC Name |
hypoboric acid | |
---|---|---|
Source | PubChem | |
URL | https://pubchem.ncbi.nlm.nih.gov | |
Description | Data deposited in or computed by PubChem | |
InChI |
InChI=1S/B2H4O4/c3-1(4)2(5)6/h3-6H | |
Source | PubChem | |
URL | https://pubchem.ncbi.nlm.nih.gov | |
Description | Data deposited in or computed by PubChem | |
InChI Key |
SKOWZLGOFVSKLB-UHFFFAOYSA-N | |
Source | PubChem | |
URL | https://pubchem.ncbi.nlm.nih.gov | |
Description | Data deposited in or computed by PubChem | |
Canonical SMILES |
B(B(O)O)(O)O | |
Source | PubChem | |
URL | https://pubchem.ncbi.nlm.nih.gov | |
Description | Data deposited in or computed by PubChem | |
Molecular Formula |
B2H4O4 | |
Source | PubChem | |
URL | https://pubchem.ncbi.nlm.nih.gov | |
Description | Data deposited in or computed by PubChem | |
DSSTOX Substance ID |
DTXSID40450702 | |
Record name | Tetrahydroxydiboron | |
Source | EPA DSSTox | |
URL | https://comptox.epa.gov/dashboard/DTXSID40450702 | |
Description | DSSTox provides a high quality public chemistry resource for supporting improved predictive toxicology. | |
Molecular Weight |
89.66 g/mol | |
Source | PubChem | |
URL | https://pubchem.ncbi.nlm.nih.gov | |
Description | Data deposited in or computed by PubChem | |
CAS No. |
13675-18-8 | |
Record name | Diboronic acid | |
Source | CAS Common Chemistry | |
URL | https://commonchemistry.cas.org/detail?cas_rn=13675-18-8 | |
Description | CAS Common Chemistry is an open community resource for accessing chemical information. Nearly 500,000 chemical substances from CAS REGISTRY cover areas of community interest, including common and frequently regulated chemicals, and those relevant to high school and undergraduate chemistry classes. This chemical information, curated by our expert scientists, is provided in alignment with our mission as a division of the American Chemical Society. | |
Explanation | The data from CAS Common Chemistry is provided under a CC-BY-NC 4.0 license, unless otherwise stated. | |
Record name | Diboron tetrahydroxide | |
Source | ChemIDplus | |
URL | https://pubchem.ncbi.nlm.nih.gov/substance/?source=chemidplus&sourceid=0013675188 | |
Description | ChemIDplus is a free, web search system that provides access to the structure and nomenclature authority files used for the identification of chemical substances cited in National Library of Medicine (NLM) databases, including the TOXNET system. | |
Record name | Tetrahydroxydiboron | |
Source | EPA DSSTox | |
URL | https://comptox.epa.gov/dashboard/DTXSID40450702 | |
Description | DSSTox provides a high quality public chemistry resource for supporting improved predictive toxicology. | |
Record name | Tetrahydroxydiborane | |
Source | European Chemicals Agency (ECHA) | |
URL | https://echa.europa.eu/information-on-chemicals | |
Description | The European Chemicals Agency (ECHA) is an agency of the European Union which is the driving force among regulatory authorities in implementing the EU's groundbreaking chemicals legislation for the benefit of human health and the environment as well as for innovation and competitiveness. | |
Explanation | Use of the information, documents and data from the ECHA website is subject to the terms and conditions of this Legal Notice, and subject to other binding limitations provided for under applicable law, the information, documents and data made available on the ECHA website may be reproduced, distributed and/or used, totally or in part, for non-commercial purposes provided that ECHA is acknowledged as the source: "Source: European Chemicals Agency, http://echa.europa.eu/". Such acknowledgement must be included in each copy of the material. ECHA permits and encourages organisations and individuals to create links to the ECHA website under the following cumulative conditions: Links can only be made to webpages that provide a link to the Legal Notice page. | |
Record name | DIBORON TETRAHYDROXIDE | |
Source | FDA Global Substance Registration System (GSRS) | |
URL | https://gsrs.ncats.nih.gov/ginas/app/beta/substances/D0Q7FO865W | |
Description | The FDA Global Substance Registration System (GSRS) enables the efficient and accurate exchange of information on what substances are in regulated products. Instead of relying on names, which vary across regulatory domains, countries, and regions, the GSRS knowledge base makes it possible for substances to be defined by standardized, scientific descriptions. | |
Explanation | Unless otherwise noted, the contents of the FDA website (www.fda.gov), both text and graphics, are not copyrighted. They are in the public domain and may be republished, reprinted and otherwise used freely by anyone without the need to obtain permission from FDA. Credit to the U.S. Food and Drug Administration as the source is appreciated but not required. | |
Retrosynthesis Analysis
AI-Powered Synthesis Planning: Our tool employs the Template_relevance Pistachio, Template_relevance Bkms_metabolic, Template_relevance Pistachio_ringbreaker, Template_relevance Reaxys, Template_relevance Reaxys_biocatalysis model, leveraging a vast database of chemical reactions to predict feasible synthetic routes.
One-Step Synthesis Focus: Specifically designed for one-step synthesis, it provides concise and direct routes for your target compounds, streamlining the synthesis process.
Accurate Predictions: Utilizing the extensive PISTACHIO, BKMS_METABOLIC, PISTACHIO_RINGBREAKER, REAXYS, REAXYS_BIOCATALYSIS database, our tool offers high-accuracy predictions, reflecting the latest in chemical research and data.
Strategy Settings
Precursor scoring | Relevance Heuristic |
---|---|
Min. plausibility | 0.01 |
Model | Template_relevance |
Template Set | Pistachio/Bkms_metabolic/Pistachio_ringbreaker/Reaxys/Reaxys_biocatalysis |
Top-N result to add to graph | 6 |
Feasible Synthetic Routes
Disclaimer and Information on In-Vitro Research Products
Please be aware that all articles and product information presented on BenchChem are intended solely for informational purposes. The products available for purchase on BenchChem are specifically designed for in-vitro studies, which are conducted outside of living organisms. In-vitro studies, derived from the Latin term "in glass," involve experiments performed in controlled laboratory settings using cells or tissues. It is important to note that these products are not categorized as medicines or drugs, and they have not received approval from the FDA for the prevention, treatment, or cure of any medical condition, ailment, or disease. We must emphasize that any form of bodily introduction of these products into humans or animals is strictly prohibited by law. It is essential to adhere to these guidelines to ensure compliance with legal and ethical standards in research and experimentation.