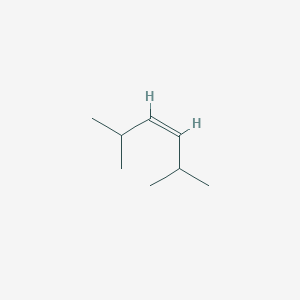
cis-2,5-Dimethyl-3-hexene
Overview
Description
cis-2,5-Dimethyl-3-hexene: is an organic compound with the molecular formula C8H16 . It is a type of alkene, characterized by the presence of a carbon-carbon double bond. The “cis” configuration indicates that the two methyl groups attached to the double-bonded carbons are on the same side of the molecule. This compound is a colorless liquid that is soluble in most organic solvents such as ether, alcohol, and benzene .
Mechanism of Action
Target of Action
cis-2,5-Dimethyl-3-hexene, also known as (Z)-2,5-Dimethylhex-3-ene, is an organic compound that belongs to the class of alkenes . The primary targets of this compound are not well-defined due to its nature as a simple alkene. Alkenes are primarily involved in chemical reactions rather than biological interactions.
Mode of Action
The mode of action of this compound is primarily through its chemical reactivity as an alkene. Alkenes are characterized by the presence of a carbon-carbon double bond, which is a region of high electron density and is therefore susceptible to attack by electrophiles . This can lead to a variety of chemical reactions, including addition, oxidation, and polymerization .
Biochemical Pathways
For example, alkenes can be oxidized to form epoxides, which are reactive compounds that can further react with various biological molecules .
Pharmacokinetics
The pharmacokinetics of this compound are not well-studied. As a small, nonpolar molecule, it is likely to be absorbed well in the gastrointestinal tract if ingested. Its volatility may also lead to significant inhalation exposure . Once in the body, it may be metabolized by various enzymes, potentially forming reactive metabolites. The compound is likely to be excreted in the urine and feces, although the exact proportions are unknown.
Action Environment
The action of this compound can be influenced by various environmental factors. For example, its reactivity as an alkene can be affected by the presence of various catalysts or inhibitors . Additionally, its volatility means that it can easily evaporate, which can lead to inhalation exposure . Environmental conditions such as temperature and humidity can also affect its stability and reactivity.
Preparation Methods
Synthetic Routes and Reaction Conditions:
Intramolecular Hydrogen Transfer Reaction: One common method for synthesizing cis-2,5-Dimethyl-3-hexene involves the intramolecular hydrogen transfer reaction of isoprene.
Hydroboration-Protonolysis: Another method involves the hydroboration of 3-hexyne followed by protonolysis.
Industrial Production Methods: Industrial production of this compound often involves the use of catalytic processes to ensure high yield and purity. The use of platinum or other transition metal catalysts is common in these processes .
Chemical Reactions Analysis
Types of Reactions:
Oxidation: cis-2,5-Dimethyl-3-hexene can undergo oxidation reactions to form epoxides and diols.
Reduction: Reduction of this compound can be achieved using hydrogenation reactions, where hydrogen gas is used in the presence of a metal catalyst to convert the double bond into a single bond.
Substitution: This compound can also undergo substitution reactions, where one of the hydrogen atoms is replaced by another atom or group of atoms.
Common Reagents and Conditions:
Oxidation: meta-chloroperoxybenzoic acid, osmium tetroxide.
Reduction: Hydrogen gas, metal catalysts such as palladium or platinum.
Substitution: Halogens, acids, or bases depending on the desired product.
Major Products:
Epoxides: Formed from oxidation reactions.
Alkanes: Formed from reduction reactions.
Substituted Alkenes: Formed from substitution reactions.
Scientific Research Applications
cis-2,5-Dimethyl-3-hexene has a variety of applications in scientific research:
Organic Synthesis: It is widely used as an intermediate in the synthesis of more complex organic compounds.
Catalysis Studies: Researchers use this compound to study catalytic processes and reaction mechanisms.
Material Science: It is used in the development of new materials with specific properties.
Chemical Kinetics: The compound is used to study reaction kinetics and mechanisms due to its well-defined structure and reactivity.
Comparison with Similar Compounds
trans-2,5-Dimethyl-3-hexene: The trans isomer has the methyl groups on opposite sides of the double bond, leading to different physical and chemical properties.
cis-2-Butene: Another cis-disubstituted alkene, but with a shorter carbon chain.
cis-1,2-Dichloroethene: A cis-disubstituted alkene with chlorine atoms instead of methyl groups.
Uniqueness: cis-2,5-Dimethyl-3-hexene is unique due to its specific molecular structure, which imparts distinct reactivity and physical properties. Its symmetrical structure and the presence of two methyl groups on the same side of the double bond make it an interesting compound for studying stereochemical effects in organic reactions .
Biological Activity
cis-2,5-Dimethyl-3-hexene is an organic compound classified as an alkene, characterized by its carbon-carbon double bond and the specific arrangement of its substituents. This compound has garnered interest in various fields, including organic synthesis and biological research, due to its unique structural properties and potential reactivity. This article delves into the biological activity of this compound, examining its mechanisms of action, potential biological interactions, and relevant research findings.
- Molecular Formula : C8H16
- Structure : The "cis" configuration indicates that the two methyl groups attached to the double-bonded carbons are on the same side.
The biological activity of this compound primarily arises from its chemical reactivity as an alkene. Alkenes are known for their susceptibility to electrophilic attack due to the high electron density at the double bond. Key mechanisms include:
- Electrophilic Addition : The double bond can react with electrophiles, leading to various products such as alcohols or halides.
- Oxidation Reactions : this compound can be oxidized to form epoxides or diols, which may interact with biological molecules and influence metabolic pathways.
- Substitution Reactions : The compound can undergo substitution reactions where hydrogen atoms are replaced by other groups, potentially altering its biological interactions.
Research Findings
Various studies have explored the reactivity and applications of this compound in scientific research:
- Organic Synthesis : It serves as an intermediate in synthesizing more complex organic compounds. Its reactivity allows for diverse synthetic pathways in organic chemistry .
- Catalysis Studies : Researchers utilize this compound to investigate catalytic processes and reaction mechanisms involving alkenes. Its well-defined structure aids in understanding stereochemical effects during reactions .
- Material Science Applications : The compound is also explored for developing new materials with specific properties due to its unique structural characteristics .
Comparative Analysis
To understand the uniqueness of this compound compared to similar compounds, a comparison table is presented below:
Compound | Structure Type | Key Properties |
---|---|---|
This compound | Alkene (cis configuration) | High reactivity due to double bond |
trans-2,5-Dimethyl-3-hexene | Alkene (trans configuration) | Different physical properties; lower reactivity |
cis-2-Hexene | Alkene (cis configuration) | Shorter carbon chain; similar reactivity |
cis-Methylcyclohexane | Cycloalkane | Saturated structure; less reactive than alkenes |
Case Study 1: Oxidative Metabolism
A study examined the oxidative metabolism of alkenes in biological systems. It was found that compounds similar to this compound could undergo enzymatic oxidation leading to reactive intermediates capable of forming adducts with cellular macromolecules .
Case Study 2: Environmental Impact
Research highlighted that alkenes like this compound might contribute to environmental toxicity when released into ecosystems. Their ability to react with various biological molecules raises concerns regarding their ecological impact .
Properties
IUPAC Name |
(Z)-2,5-dimethylhex-3-ene | |
---|---|---|
Source | PubChem | |
URL | https://pubchem.ncbi.nlm.nih.gov | |
Description | Data deposited in or computed by PubChem | |
InChI |
InChI=1S/C8H16/c1-7(2)5-6-8(3)4/h5-8H,1-4H3/b6-5- | |
Source | PubChem | |
URL | https://pubchem.ncbi.nlm.nih.gov | |
Description | Data deposited in or computed by PubChem | |
InChI Key |
KNCMKWVOMRUHKZ-WAYWQWQTSA-N | |
Source | PubChem | |
URL | https://pubchem.ncbi.nlm.nih.gov | |
Description | Data deposited in or computed by PubChem | |
Canonical SMILES |
CC(C)C=CC(C)C | |
Source | PubChem | |
URL | https://pubchem.ncbi.nlm.nih.gov | |
Description | Data deposited in or computed by PubChem | |
Isomeric SMILES |
CC(C)/C=C\C(C)C | |
Source | PubChem | |
URL | https://pubchem.ncbi.nlm.nih.gov | |
Description | Data deposited in or computed by PubChem | |
Molecular Formula |
C8H16 | |
Source | PubChem | |
URL | https://pubchem.ncbi.nlm.nih.gov | |
Description | Data deposited in or computed by PubChem | |
Molecular Weight |
112.21 g/mol | |
Source | PubChem | |
URL | https://pubchem.ncbi.nlm.nih.gov | |
Description | Data deposited in or computed by PubChem | |
CAS No. |
10557-44-5 | |
Record name | 3-Hexene, 2,5-dimethyl-, (Z)- | |
Source | ChemIDplus | |
URL | https://pubchem.ncbi.nlm.nih.gov/substance/?source=chemidplus&sourceid=0010557445 | |
Description | ChemIDplus is a free, web search system that provides access to the structure and nomenclature authority files used for the identification of chemical substances cited in National Library of Medicine (NLM) databases, including the TOXNET system. | |
Retrosynthesis Analysis
AI-Powered Synthesis Planning: Our tool employs the Template_relevance Pistachio, Template_relevance Bkms_metabolic, Template_relevance Pistachio_ringbreaker, Template_relevance Reaxys, Template_relevance Reaxys_biocatalysis model, leveraging a vast database of chemical reactions to predict feasible synthetic routes.
One-Step Synthesis Focus: Specifically designed for one-step synthesis, it provides concise and direct routes for your target compounds, streamlining the synthesis process.
Accurate Predictions: Utilizing the extensive PISTACHIO, BKMS_METABOLIC, PISTACHIO_RINGBREAKER, REAXYS, REAXYS_BIOCATALYSIS database, our tool offers high-accuracy predictions, reflecting the latest in chemical research and data.
Strategy Settings
Precursor scoring | Relevance Heuristic |
---|---|
Min. plausibility | 0.01 |
Model | Template_relevance |
Template Set | Pistachio/Bkms_metabolic/Pistachio_ringbreaker/Reaxys/Reaxys_biocatalysis |
Top-N result to add to graph | 6 |
Feasible Synthetic Routes
Disclaimer and Information on In-Vitro Research Products
Please be aware that all articles and product information presented on BenchChem are intended solely for informational purposes. The products available for purchase on BenchChem are specifically designed for in-vitro studies, which are conducted outside of living organisms. In-vitro studies, derived from the Latin term "in glass," involve experiments performed in controlled laboratory settings using cells or tissues. It is important to note that these products are not categorized as medicines or drugs, and they have not received approval from the FDA for the prevention, treatment, or cure of any medical condition, ailment, or disease. We must emphasize that any form of bodily introduction of these products into humans or animals is strictly prohibited by law. It is essential to adhere to these guidelines to ensure compliance with legal and ethical standards in research and experimentation.