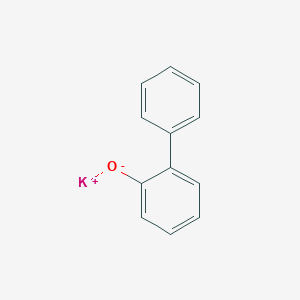
o-Phenylphenol potassium salt
Overview
Description
o-Phenylphenol potassium salt, also known as potassium o-phenylphenate, is an organic compound derived from o-phenylphenol. It is commonly used as a preservative and fungicide due to its antimicrobial properties. The compound is a white to slightly yellow crystalline powder that is soluble in water.
Preparation Methods
Synthetic Routes and Reaction Conditions
The preparation of o-Phenylphenol potassium salt typically involves the neutralization of o-phenylphenol with potassium hydroxide. The reaction can be represented as follows:
C12H10O+KOH→C12H9OK+H2O
In this reaction, o-phenylphenol (C_{12}H_{10}O) reacts with potassium hydroxide (KOH) to form this compound (C_{12}H_{9}OK) and water (H_{2}O). The reaction is usually carried out in an aqueous medium at room temperature.
Industrial Production Methods
Industrial production of this compound involves the same neutralization reaction but on a larger scale. The process includes dissolving o-phenylphenol in an organic solvent, followed by the addition of potassium hydroxide solution. The product is then separated, filtered, and dried to obtain the final compound .
Chemical Reactions Analysis
Types of Reactions
o-Phenylphenol potassium salt undergoes various chemical reactions, including:
Oxidation: It can be oxidized to form quinones.
Reduction: It can be reduced to form hydroquinones.
Electrophilic Aromatic Substitution: The compound is highly reactive towards electrophilic aromatic substitution due to the activating effect of the hydroxyl group.
Common Reagents and Conditions
Oxidation: Common oxidizing agents include sodium dichromate, chromium trioxide, and potassium nitrosodisulfonate.
Reduction: Reducing agents such as sodium borohydride can be used.
Electrophilic Aromatic Substitution: Reactions typically involve reagents like bromine, chlorine, and nitric acid under acidic conditions.
Major Products Formed
Oxidation: Quinones
Reduction: Hydroquinones
Electrophilic Aromatic Substitution: Substituted phenols
Scientific Research Applications
o-Phenylphenol potassium salt has a wide range of applications in scientific research:
Chemistry: Used as a reagent in various organic synthesis reactions.
Biology: Employed as a preservative in biological samples to prevent microbial contamination.
Medicine: Investigated for its antimicrobial properties and potential use in disinfectants.
Industry: Utilized as a preservative in cosmetics, food packaging, and agricultural products.
Mechanism of Action
The antimicrobial action of o-Phenylphenol potassium salt is primarily due to its ability to disrupt microbial cell membranes. The compound interacts with the lipid bilayer of the cell membrane, leading to increased permeability and leakage of cellular contents. This ultimately results in cell death. The molecular targets include membrane proteins and lipids, and the pathways involved are related to membrane integrity and function .
Comparison with Similar Compounds
Similar Compounds
- Sodium o-phenylphenate
- MEA o-phenylphenate
- o-Phenylphenol
Uniqueness
Compared to its sodium and MEA counterparts, o-Phenylphenol potassium salt has higher water solubility, which can enhance its effectiveness in aqueous applications. Additionally, it is less corrosive to the skin compared to sodium o-phenylphenate, making it safer for use in certain applications .
Properties
CAS No. |
13707-65-8 |
---|---|
Molecular Formula |
C12H10KO |
Molecular Weight |
209.30 g/mol |
IUPAC Name |
potassium;2-phenylphenolate |
InChI |
InChI=1S/C12H10O.K/c13-12-9-5-4-8-11(12)10-6-2-1-3-7-10;/h1-9,13H; |
InChI Key |
LEUKGHVZJCKWMI-UHFFFAOYSA-N |
Isomeric SMILES |
C1=CC=C(C=C1)C2=CC=CC=C2[O-].[K+] |
SMILES |
C1=CC=C(C=C1)C2=CC=CC=C2[O-].[K+] |
Canonical SMILES |
C1=CC=C(C=C1)C2=CC=CC=C2O.[K] |
13707-65-8 | |
Pictograms |
Corrosive; Irritant |
Origin of Product |
United States |
Synthesis routes and methods
Procedure details
Retrosynthesis Analysis
AI-Powered Synthesis Planning: Our tool employs the Template_relevance Pistachio, Template_relevance Bkms_metabolic, Template_relevance Pistachio_ringbreaker, Template_relevance Reaxys, Template_relevance Reaxys_biocatalysis model, leveraging a vast database of chemical reactions to predict feasible synthetic routes.
One-Step Synthesis Focus: Specifically designed for one-step synthesis, it provides concise and direct routes for your target compounds, streamlining the synthesis process.
Accurate Predictions: Utilizing the extensive PISTACHIO, BKMS_METABOLIC, PISTACHIO_RINGBREAKER, REAXYS, REAXYS_BIOCATALYSIS database, our tool offers high-accuracy predictions, reflecting the latest in chemical research and data.
Strategy Settings
Precursor scoring | Relevance Heuristic |
---|---|
Min. plausibility | 0.01 |
Model | Template_relevance |
Template Set | Pistachio/Bkms_metabolic/Pistachio_ringbreaker/Reaxys/Reaxys_biocatalysis |
Top-N result to add to graph | 6 |
Feasible Synthetic Routes
Disclaimer and Information on In-Vitro Research Products
Please be aware that all articles and product information presented on BenchChem are intended solely for informational purposes. The products available for purchase on BenchChem are specifically designed for in-vitro studies, which are conducted outside of living organisms. In-vitro studies, derived from the Latin term "in glass," involve experiments performed in controlled laboratory settings using cells or tissues. It is important to note that these products are not categorized as medicines or drugs, and they have not received approval from the FDA for the prevention, treatment, or cure of any medical condition, ailment, or disease. We must emphasize that any form of bodily introduction of these products into humans or animals is strictly prohibited by law. It is essential to adhere to these guidelines to ensure compliance with legal and ethical standards in research and experimentation.