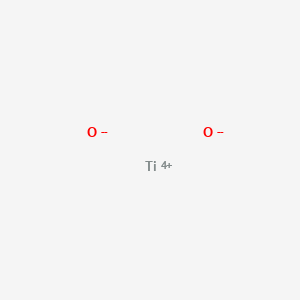
Trititanium oxide
Overview
Description
Preparation Methods
Synthetic Routes and Reaction Conditions: Trititanium oxide can be synthesized through various methods, including solid-state reactions, chemical vapor deposition, and hydrothermal synthesis. One common method involves the reduction of titanium dioxide with titanium metal at high temperatures. The reaction typically occurs at around 1600°C, resulting in the formation of this compound .
Industrial Production Methods: In industrial settings, this compound is often produced using the reduction of titanium dioxide with hydrogen gas. This process involves heating titanium dioxide in a hydrogen atmosphere at elevated temperatures, leading to the formation of this compound. The reaction conditions, such as temperature and pressure, are carefully controlled to ensure the desired phase and purity of the product .
Chemical Reactions Analysis
Types of Reactions: Trititanium oxide undergoes various chemical reactions, including oxidation, reduction, and substitution reactions. It is known for its ability to participate in redox reactions, making it a valuable material in catalysis and energy storage applications .
Common Reagents and Conditions:
Oxidation: this compound can be oxidized to higher oxidation states using oxidizing agents such as oxygen or hydrogen peroxide. The reaction typically occurs at elevated temperatures.
Reduction: Reduction of this compound can be achieved using reducing agents like hydrogen gas or carbon monoxide. This process is often carried out at high temperatures.
Major Products Formed: The major products formed from these reactions depend on the specific reagents and conditions used. For example, oxidation of this compound can yield higher oxides of titanium, while reduction can produce lower oxides or metallic titanium .
Scientific Research Applications
Trititanium oxide has a wide range of scientific research applications, including:
Chemistry: this compound is used as a catalyst in various chemical reactions, including oxidation and reduction processes.
Biology: In biological research, this compound is explored for its potential use in biosensors and bioimaging.
Medicine: this compound is investigated for its potential use in drug delivery systems and medical implants.
Industry: In industrial applications, this compound is used in the production of advanced materials, such as ceramics and coatings.
Mechanism of Action
The mechanism of action of trititanium oxide involves its ability to undergo redox reactions, which are crucial for its catalytic and energy storage applications. The compound can facilitate electron transfer processes, making it effective in catalysis and photocatalysis. The molecular targets and pathways involved in these reactions depend on the specific application and reaction conditions .
Comparison with Similar Compounds
Titanium dioxide (TiO₂): Titanium dioxide is a widely studied compound with applications in photocatalysis, pigments, and sunscreens.
Titanium(III) oxide (Ti₂O₃): Titanium(III) oxide is another titanium oxide with distinct properties.
Titanium monoxide (TiO): Titanium monoxide is a less common oxide of titanium with unique properties.
Uniqueness of Trititanium Oxide: this compound stands out due to its multiple crystalline forms and phase transition properties. The reversible phase transitions between different forms of this compound offer unique opportunities for applications in data storage and energy conversion. Additionally, its ability to undergo redox reactions makes it a versatile material for catalysis and environmental applications .
Properties
IUPAC Name |
oxygen(2-);titanium(4+) | |
---|---|---|
Details | Computed by Lexichem TK 2.7.0 (PubChem release 2021.05.07) | |
Source | PubChem | |
URL | https://pubchem.ncbi.nlm.nih.gov | |
Description | Data deposited in or computed by PubChem | |
InChI |
InChI=1S/2O.Ti/q2*-2;+4 | |
Details | Computed by InChI 1.0.6 (PubChem release 2021.05.07) | |
Source | PubChem | |
URL | https://pubchem.ncbi.nlm.nih.gov | |
Description | Data deposited in or computed by PubChem | |
InChI Key |
SOQBVABWOPYFQZ-UHFFFAOYSA-N | |
Details | Computed by InChI 1.0.6 (PubChem release 2021.05.07) | |
Source | PubChem | |
URL | https://pubchem.ncbi.nlm.nih.gov | |
Description | Data deposited in or computed by PubChem | |
Canonical SMILES |
[O-2].[O-2].[Ti+4] | |
Details | Computed by OEChem 2.3.0 (PubChem release 2021.05.07) | |
Source | PubChem | |
URL | https://pubchem.ncbi.nlm.nih.gov | |
Description | Data deposited in or computed by PubChem | |
Molecular Formula |
O2Ti | |
Details | Computed by PubChem 2.1 (PubChem release 2021.05.07) | |
Source | PubChem | |
URL | https://pubchem.ncbi.nlm.nih.gov | |
Description | Data deposited in or computed by PubChem | |
DSSTOX Substance ID |
DTXSID701015366 | |
Record name | Titanium(4+) dioxide | |
Source | EPA DSSTox | |
URL | https://comptox.epa.gov/dashboard/DTXSID701015366 | |
Description | DSSTox provides a high quality public chemistry resource for supporting improved predictive toxicology. | |
Molecular Weight |
79.866 g/mol | |
Details | Computed by PubChem 2.1 (PubChem release 2021.08.13) | |
Source | PubChem | |
URL | https://pubchem.ncbi.nlm.nih.gov | |
Description | Data deposited in or computed by PubChem | |
CAS No. |
12035-95-9, 51745-87-0, 13463-67-7 | |
Record name | Titanium oxide (Ti3O) | |
Source | ChemIDplus | |
URL | https://pubchem.ncbi.nlm.nih.gov/substance/?source=chemidplus&sourceid=0012035959 | |
Description | ChemIDplus is a free, web search system that provides access to the structure and nomenclature authority files used for the identification of chemical substances cited in National Library of Medicine (NLM) databases, including the TOXNET system. | |
Record name | Titanium oxide | |
Source | ChemIDplus | |
URL | https://pubchem.ncbi.nlm.nih.gov/substance/?source=chemidplus&sourceid=0051745870 | |
Description | ChemIDplus is a free, web search system that provides access to the structure and nomenclature authority files used for the identification of chemical substances cited in National Library of Medicine (NLM) databases, including the TOXNET system. | |
Record name | Titanium oxide (Ti3O) | |
Source | EPA Chemicals under the TSCA | |
URL | https://www.epa.gov/chemicals-under-tsca | |
Description | EPA Chemicals under the Toxic Substances Control Act (TSCA) collection contains information on chemicals and their regulations under TSCA, including non-confidential content from the TSCA Chemical Substance Inventory and Chemical Data Reporting. | |
Record name | Titanium(4+) dioxide | |
Source | EPA DSSTox | |
URL | https://comptox.epa.gov/dashboard/DTXSID701015366 | |
Description | DSSTox provides a high quality public chemistry resource for supporting improved predictive toxicology. | |
Record name | Trititanium oxide | |
Source | European Chemicals Agency (ECHA) | |
URL | https://echa.europa.eu/substance-information/-/substanceinfo/100.031.654 | |
Description | The European Chemicals Agency (ECHA) is an agency of the European Union which is the driving force among regulatory authorities in implementing the EU's groundbreaking chemicals legislation for the benefit of human health and the environment as well as for innovation and competitiveness. | |
Explanation | Use of the information, documents and data from the ECHA website is subject to the terms and conditions of this Legal Notice, and subject to other binding limitations provided for under applicable law, the information, documents and data made available on the ECHA website may be reproduced, distributed and/or used, totally or in part, for non-commercial purposes provided that ECHA is acknowledged as the source: "Source: European Chemicals Agency, http://echa.europa.eu/". Such acknowledgement must be included in each copy of the material. ECHA permits and encourages organisations and individuals to create links to the ECHA website under the following cumulative conditions: Links can only be made to webpages that provide a link to the Legal Notice page. | |
Record name | TITANIUM DIOXIDE | |
Source | FDA Global Substance Registration System (GSRS) | |
URL | https://gsrs.ncats.nih.gov/ginas/app/beta/substances/15FIX9V2JP | |
Description | The FDA Global Substance Registration System (GSRS) enables the efficient and accurate exchange of information on what substances are in regulated products. Instead of relying on names, which vary across regulatory domains, countries, and regions, the GSRS knowledge base makes it possible for substances to be defined by standardized, scientific descriptions. | |
Explanation | Unless otherwise noted, the contents of the FDA website (www.fda.gov), both text and graphics, are not copyrighted. They are in the public domain and may be republished, reprinted and otherwise used freely by anyone without the need to obtain permission from FDA. Credit to the U.S. Food and Drug Administration as the source is appreciated but not required. | |
Synthesis routes and methods I
Procedure details
Synthesis routes and methods II
Procedure details
Synthesis routes and methods III
Procedure details
Synthesis routes and methods IV
Procedure details
Disclaimer and Information on In-Vitro Research Products
Please be aware that all articles and product information presented on BenchChem are intended solely for informational purposes. The products available for purchase on BenchChem are specifically designed for in-vitro studies, which are conducted outside of living organisms. In-vitro studies, derived from the Latin term "in glass," involve experiments performed in controlled laboratory settings using cells or tissues. It is important to note that these products are not categorized as medicines or drugs, and they have not received approval from the FDA for the prevention, treatment, or cure of any medical condition, ailment, or disease. We must emphasize that any form of bodily introduction of these products into humans or animals is strictly prohibited by law. It is essential to adhere to these guidelines to ensure compliance with legal and ethical standards in research and experimentation.