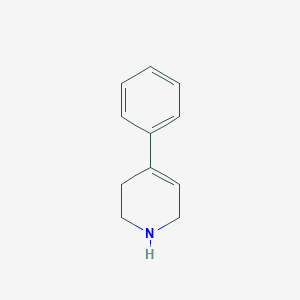
4-Phenyl-1,2,3,6-tetrahydropyridine
Overview
Description
4-Phenyl-1,2,3,6-tetrahydropyridine is an organic compound classified as a tetrahydropyridine. It is of significant interest due to its role as a precursor to the neurotoxin 1-methyl-4-phenylpyridinium, which is known to cause symptoms of Parkinson’s disease by destroying dopaminergic neurons in the brain .
Mechanism of Action
Target of Action
The primary target of 4-Phenyl-1,2,3,6-tetrahydropyridine is the dopaminergic neurons in the brain . It has been found to have an inhibitory potential against poly (ADP-ribose) polymerase-1 (PARP-1) .
Mode of Action
This compound is a lipophilic compound that can cross the blood-brain barrier . Once inside the brain, it is metabolized into the toxic cation 1-methyl-4-phenylpyridinium (MPP+) . This metabolite interferes with the function of dopaminergic neurons, leading to their destruction .
Pharmacokinetics
It is known that the compound is lipophilic, allowing it to cross the blood-brain barrier . Once in the brain, it is metabolized to MPP+ .
Result of Action
The action of this compound results in the destruction of dopaminergic neurons in the brain, leading to symptoms similar to those seen in Parkinson’s disease . The presence of the this compound fragment improves the inhibitory potential of benzamide analogs against PARP-1 .
Action Environment
The action of this compound can be influenced by various environmental factors. For example, the presence of monoamine oxidase inhibitors (MAOIs) can prevent the metabolism of the compound to MPP+, minimizing its toxicity and preventing neural death
Biochemical Analysis
Biochemical Properties
4-Phenyl-1,2,3,6-tetrahydropyridine is known to interact with various enzymes and proteins. It is metabolized to 1-methyl-4-phenylpyridine (MPP+), which can cause free radical production in vivo and lead to oxidative stress . The presence of the this compound fragment improves the inhibitory potential of benzamide analogs against poly (ADP-ribose) polymerase-1 (PARP-1) .
Cellular Effects
The cellular effects of this compound are primarily observed in dopaminergic neurons. It has been shown to cause inflammation, excitotoxicity, mitochondrial apoptosis, formation of inclusion bodies, and oxidative stress that leads to dopaminergic neuronal damage in the striatum and substantia nigra .
Molecular Mechanism
The molecular mechanism of this compound involves its metabolism to MPP+ by the enzyme monoamine oxidase B (MAO-B) of glial cells, specifically astrocytes . MPP+ interferes with complex I of the electron transport chain, a component of mitochondrial metabolism, which leads to cell death and causes the buildup of free radicals .
Temporal Effects in Laboratory Settings
It is known that MPTP-induced motor and non-motor dysfunction were ameliorated with glimepiride post-treatment .
Dosage Effects in Animal Models
The effects of this compound vary with different dosages in animal models. For instance, MPTP at a dosage of 25 mg/kg, followed by probenecid (250 mg/kg), was administered via i.p. injection for five consecutive days to induce a mouse model of Parkinson’s disease .
Metabolic Pathways
The metabolic pathway of this compound involves its conversion to MPP+ by the enzyme MAO-B . This process is critical in the induction of Parkinson-like symptoms.
Transport and Distribution
This compound is a lipophilic compound and can therefore cross the blood–brain barrier . Once inside the brain, it is metabolized into MPP+ by the enzyme MAO-B of glial cells .
Subcellular Localization
Its metabolite MPP+ is known to primarily affect dopamine-producing neurons in a part of the brain called the pars compacta of the substantia nigra .
Preparation Methods
Synthetic Routes and Reaction Conditions
4-Phenyl-1,2,3,6-tetrahydropyridine can be synthesized through various methods. One common route involves the reaction of phenylacetonitrile with formaldehyde and ammonium acetate under acidic conditions. The reaction typically proceeds through a Mannich reaction followed by cyclization to form the tetrahydropyridine ring .
Industrial Production Methods
Industrial production of this compound often involves similar synthetic routes but on a larger scale. The reaction conditions are optimized to ensure high yield and purity of the product. The compound is usually purified through recrystallization or distillation .
Chemical Reactions Analysis
Types of Reactions
4-Phenyl-1,2,3,6-tetrahydropyridine undergoes various chemical reactions, including:
Oxidation: It can be oxidized to form 1-methyl-4-phenylpyridinium, a neurotoxic compound.
Reduction: The compound can be reduced to form this compound derivatives.
Substitution: It can undergo substitution reactions, particularly at the nitrogen atom.
Common Reagents and Conditions
Oxidation: Common oxidizing agents include potassium permanganate and hydrogen peroxide.
Reduction: Reducing agents such as lithium aluminum hydride are often used.
Substitution: Substitution reactions typically involve nucleophiles like alkyl halides.
Major Products Formed
Oxidation: 1-Methyl-4-phenylpyridinium
Reduction: Various this compound derivatives
Substitution: Substituted tetrahydropyridine compounds
Scientific Research Applications
4-Phenyl-1,2,3,6-tetrahydropyridine has several scientific research applications:
Chemistry: It is used as a precursor in the synthesis of various organic compounds.
Biology: The compound is used to study the mechanisms of neurodegeneration.
Medicine: It is employed in research related to Parkinson’s disease to understand the disease’s progression and potential treatments.
Industry: The compound is used in the production of pharmaceuticals and other chemical products.
Comparison with Similar Compounds
4-Phenyl-1,2,3,6-tetrahydropyridine is unique due to its specific neurotoxic effects. Similar compounds include:
1-Methyl-4-phenyl-1,2,3,6-tetrahydropyridine: Also known for its neurotoxic properties.
6-Hydroxydopamine: Another neurotoxin used in Parkinson’s disease research.
Rotenone: A naturally occurring compound that also induces Parkinson-like symptoms.
These compounds are used in research to model Parkinson’s disease and study neurodegeneration, but this compound is particularly notable for its specific mechanism of action and effects on dopaminergic neurons .
Properties
IUPAC Name |
4-phenyl-1,2,3,6-tetrahydropyridine | |
---|---|---|
Source | PubChem | |
URL | https://pubchem.ncbi.nlm.nih.gov | |
Description | Data deposited in or computed by PubChem | |
InChI |
InChI=1S/C11H13N/c1-2-4-10(5-3-1)11-6-8-12-9-7-11/h1-6,12H,7-9H2 | |
Source | PubChem | |
URL | https://pubchem.ncbi.nlm.nih.gov | |
Description | Data deposited in or computed by PubChem | |
InChI Key |
OMPXTQYWYRWWPH-UHFFFAOYSA-N | |
Source | PubChem | |
URL | https://pubchem.ncbi.nlm.nih.gov | |
Description | Data deposited in or computed by PubChem | |
Canonical SMILES |
C1CNCC=C1C2=CC=CC=C2 | |
Source | PubChem | |
URL | https://pubchem.ncbi.nlm.nih.gov | |
Description | Data deposited in or computed by PubChem | |
Molecular Formula |
C11H13N | |
Source | PubChem | |
URL | https://pubchem.ncbi.nlm.nih.gov | |
Description | Data deposited in or computed by PubChem | |
Related CAS |
43064-12-6 (hydrochloride) | |
Record name | 4-Phenyl-1,2,3,6-tetrahydropyridine | |
Source | ChemIDplus | |
URL | https://pubchem.ncbi.nlm.nih.gov/substance/?source=chemidplus&sourceid=0010338699 | |
Description | ChemIDplus is a free, web search system that provides access to the structure and nomenclature authority files used for the identification of chemical substances cited in National Library of Medicine (NLM) databases, including the TOXNET system. | |
DSSTOX Substance ID |
DTXSID2065040 | |
Record name | 1,2,3,6-Tetrahydro-4-phenylpyridine | |
Source | EPA DSSTox | |
URL | https://comptox.epa.gov/dashboard/DTXSID2065040 | |
Description | DSSTox provides a high quality public chemistry resource for supporting improved predictive toxicology. | |
Molecular Weight |
159.23 g/mol | |
Source | PubChem | |
URL | https://pubchem.ncbi.nlm.nih.gov | |
Description | Data deposited in or computed by PubChem | |
CAS No. |
10338-69-9 | |
Record name | 1,2,3,6-Tetrahydro-4-phenylpyridine | |
Source | CAS Common Chemistry | |
URL | https://commonchemistry.cas.org/detail?cas_rn=10338-69-9 | |
Description | CAS Common Chemistry is an open community resource for accessing chemical information. Nearly 500,000 chemical substances from CAS REGISTRY cover areas of community interest, including common and frequently regulated chemicals, and those relevant to high school and undergraduate chemistry classes. This chemical information, curated by our expert scientists, is provided in alignment with our mission as a division of the American Chemical Society. | |
Explanation | The data from CAS Common Chemistry is provided under a CC-BY-NC 4.0 license, unless otherwise stated. | |
Record name | 4-Phenyl-1,2,3,6-tetrahydropyridine | |
Source | ChemIDplus | |
URL | https://pubchem.ncbi.nlm.nih.gov/substance/?source=chemidplus&sourceid=0010338699 | |
Description | ChemIDplus is a free, web search system that provides access to the structure and nomenclature authority files used for the identification of chemical substances cited in National Library of Medicine (NLM) databases, including the TOXNET system. | |
Record name | 10338-69-9 | |
Source | DTP/NCI | |
URL | https://dtp.cancer.gov/dtpstandard/servlet/dwindex?searchtype=NSC&outputformat=html&searchlist=54451 | |
Description | The NCI Development Therapeutics Program (DTP) provides services and resources to the academic and private-sector research communities worldwide to facilitate the discovery and development of new cancer therapeutic agents. | |
Explanation | Unless otherwise indicated, all text within NCI products is free of copyright and may be reused without our permission. Credit the National Cancer Institute as the source. | |
Record name | Pyridine, 1,2,3,6-tetrahydro-4-phenyl- | |
Source | EPA Chemicals under the TSCA | |
URL | https://www.epa.gov/chemicals-under-tsca | |
Description | EPA Chemicals under the Toxic Substances Control Act (TSCA) collection contains information on chemicals and their regulations under TSCA, including non-confidential content from the TSCA Chemical Substance Inventory and Chemical Data Reporting. | |
Record name | 1,2,3,6-Tetrahydro-4-phenylpyridine | |
Source | EPA DSSTox | |
URL | https://comptox.epa.gov/dashboard/DTXSID2065040 | |
Description | DSSTox provides a high quality public chemistry resource for supporting improved predictive toxicology. | |
Record name | 10338-69-9 | |
Source | European Chemicals Agency (ECHA) | |
URL | https://echa.europa.eu/information-on-chemicals | |
Description | The European Chemicals Agency (ECHA) is an agency of the European Union which is the driving force among regulatory authorities in implementing the EU's groundbreaking chemicals legislation for the benefit of human health and the environment as well as for innovation and competitiveness. | |
Explanation | Use of the information, documents and data from the ECHA website is subject to the terms and conditions of this Legal Notice, and subject to other binding limitations provided for under applicable law, the information, documents and data made available on the ECHA website may be reproduced, distributed and/or used, totally or in part, for non-commercial purposes provided that ECHA is acknowledged as the source: "Source: European Chemicals Agency, http://echa.europa.eu/". Such acknowledgement must be included in each copy of the material. ECHA permits and encourages organisations and individuals to create links to the ECHA website under the following cumulative conditions: Links can only be made to webpages that provide a link to the Legal Notice page. | |
Synthesis routes and methods I
Procedure details
Synthesis routes and methods II
Procedure details
Retrosynthesis Analysis
AI-Powered Synthesis Planning: Our tool employs the Template_relevance Pistachio, Template_relevance Bkms_metabolic, Template_relevance Pistachio_ringbreaker, Template_relevance Reaxys, Template_relevance Reaxys_biocatalysis model, leveraging a vast database of chemical reactions to predict feasible synthetic routes.
One-Step Synthesis Focus: Specifically designed for one-step synthesis, it provides concise and direct routes for your target compounds, streamlining the synthesis process.
Accurate Predictions: Utilizing the extensive PISTACHIO, BKMS_METABOLIC, PISTACHIO_RINGBREAKER, REAXYS, REAXYS_BIOCATALYSIS database, our tool offers high-accuracy predictions, reflecting the latest in chemical research and data.
Strategy Settings
Precursor scoring | Relevance Heuristic |
---|---|
Min. plausibility | 0.01 |
Model | Template_relevance |
Template Set | Pistachio/Bkms_metabolic/Pistachio_ringbreaker/Reaxys/Reaxys_biocatalysis |
Top-N result to add to graph | 6 |
Feasible Synthetic Routes
Disclaimer and Information on In-Vitro Research Products
Please be aware that all articles and product information presented on BenchChem are intended solely for informational purposes. The products available for purchase on BenchChem are specifically designed for in-vitro studies, which are conducted outside of living organisms. In-vitro studies, derived from the Latin term "in glass," involve experiments performed in controlled laboratory settings using cells or tissues. It is important to note that these products are not categorized as medicines or drugs, and they have not received approval from the FDA for the prevention, treatment, or cure of any medical condition, ailment, or disease. We must emphasize that any form of bodily introduction of these products into humans or animals is strictly prohibited by law. It is essential to adhere to these guidelines to ensure compliance with legal and ethical standards in research and experimentation.