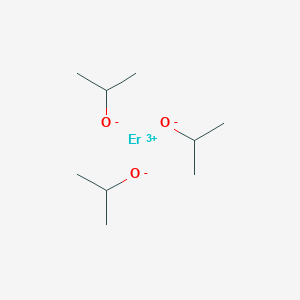
Erbium(Iii) Isopropoxide
Overview
Description
Erbium(III) isopropoxide is an organometallic compound with the chemical formula Er(OCH(CH₃)₂)₃. It is a pink powder that is highly sensitive to moisture and air. This compound is primarily used as a precursor for the synthesis of other erbium-containing compounds and materials, particularly in the field of optical fibers and other advanced materials.
Mechanism of Action
Target of Action
Erbium(III) isopropoxide is a chemical compound with the formula Er(OCH(CH3)2)3 It is known to be a precursor for aluminum-erbium complexes used in active optical fibers .
Mode of Action
As a precursor for aluminum-erbium complexes, it likely undergoes chemical reactions to form these complexes, which are then used in the production of active optical fibers .
Biochemical Pathways
Its role as a precursor in the formation of aluminum-erbium complexes suggests it may be involved in the biochemical processes related to the production and function of optical fibers .
Result of Action
Its primary known application is as a precursor in the formation of aluminum-erbium complexes for active optical fibers . The resulting complexes are likely to have specific optical properties that make them useful in this application.
Preparation Methods
Synthetic Routes and Reaction Conditions: Erbium(III) isopropoxide can be synthesized through the reaction of erbium(III) chloride with isopropanol in the presence of a base. The reaction typically proceeds as follows:
ErCl3+3(CH3
Properties
IUPAC Name |
erbium(3+);propan-2-olate | |
---|---|---|
Source | PubChem | |
URL | https://pubchem.ncbi.nlm.nih.gov | |
Description | Data deposited in or computed by PubChem | |
InChI |
InChI=1S/3C3H7O.Er/c3*1-3(2)4;/h3*3H,1-2H3;/q3*-1;+3 | |
Source | PubChem | |
URL | https://pubchem.ncbi.nlm.nih.gov | |
Description | Data deposited in or computed by PubChem | |
InChI Key |
VOCNVTAXVORJBI-UHFFFAOYSA-N | |
Source | PubChem | |
URL | https://pubchem.ncbi.nlm.nih.gov | |
Description | Data deposited in or computed by PubChem | |
Canonical SMILES |
CC(C)[O-].CC(C)[O-].CC(C)[O-].[Er+3] | |
Source | PubChem | |
URL | https://pubchem.ncbi.nlm.nih.gov | |
Description | Data deposited in or computed by PubChem | |
Molecular Formula |
C9H21ErO3 | |
Source | PubChem | |
URL | https://pubchem.ncbi.nlm.nih.gov | |
Description | Data deposited in or computed by PubChem | |
DSSTOX Substance ID |
DTXSID80370096 | |
Record name | Erbium(Iii) Isopropoxide | |
Source | EPA DSSTox | |
URL | https://comptox.epa.gov/dashboard/DTXSID80370096 | |
Description | DSSTox provides a high quality public chemistry resource for supporting improved predictive toxicology. | |
Molecular Weight |
344.52 g/mol | |
Source | PubChem | |
URL | https://pubchem.ncbi.nlm.nih.gov | |
Description | Data deposited in or computed by PubChem | |
CAS No. |
14814-07-4 | |
Record name | Erbium(Iii) Isopropoxide | |
Source | EPA DSSTox | |
URL | https://comptox.epa.gov/dashboard/DTXSID80370096 | |
Description | DSSTox provides a high quality public chemistry resource for supporting improved predictive toxicology. | |
Retrosynthesis Analysis
AI-Powered Synthesis Planning: Our tool employs the Template_relevance Pistachio, Template_relevance Bkms_metabolic, Template_relevance Pistachio_ringbreaker, Template_relevance Reaxys, Template_relevance Reaxys_biocatalysis model, leveraging a vast database of chemical reactions to predict feasible synthetic routes.
One-Step Synthesis Focus: Specifically designed for one-step synthesis, it provides concise and direct routes for your target compounds, streamlining the synthesis process.
Accurate Predictions: Utilizing the extensive PISTACHIO, BKMS_METABOLIC, PISTACHIO_RINGBREAKER, REAXYS, REAXYS_BIOCATALYSIS database, our tool offers high-accuracy predictions, reflecting the latest in chemical research and data.
Strategy Settings
Precursor scoring | Relevance Heuristic |
---|---|
Min. plausibility | 0.01 |
Model | Template_relevance |
Template Set | Pistachio/Bkms_metabolic/Pistachio_ringbreaker/Reaxys/Reaxys_biocatalysis |
Top-N result to add to graph | 6 |
Feasible Synthetic Routes
Disclaimer and Information on In-Vitro Research Products
Please be aware that all articles and product information presented on BenchChem are intended solely for informational purposes. The products available for purchase on BenchChem are specifically designed for in-vitro studies, which are conducted outside of living organisms. In-vitro studies, derived from the Latin term "in glass," involve experiments performed in controlled laboratory settings using cells or tissues. It is important to note that these products are not categorized as medicines or drugs, and they have not received approval from the FDA for the prevention, treatment, or cure of any medical condition, ailment, or disease. We must emphasize that any form of bodily introduction of these products into humans or animals is strictly prohibited by law. It is essential to adhere to these guidelines to ensure compliance with legal and ethical standards in research and experimentation.