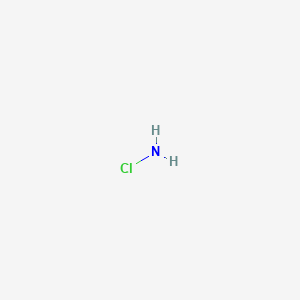
Chloramine
Overview
Description
Chloramine is a class of chemical compounds that contain chlorine and ammonia. It is primarily used as a disinfectant in water treatment processes. Chloramines are formed when ammonia is added to chlorine, and they provide longer-lasting disinfection as water moves through pipes to consumers . The three main types of inorganic chloramines are monothis compound, dithis compound, and nitrogen trichloride .
Preparation Methods
Synthetic Routes and Reaction Conditions: Chloramine is typically prepared by reacting chlorine with ammonia. The reaction can be represented as: [ \text{NH}_3 + \text{HOCl} \rightarrow \text{NH}_2\text{Cl} + \text{H}_2\text{O} ] This reaction produces monothis compound, which is the most commonly used form in water treatment .
Industrial Production Methods: In industrial settings, this compound is produced by adding anhydrous or aqueous ammonia to chlorine. The process is pH and concentration-dependent, with optimal conditions being a pH level below 7.5 and a chlorine-to-ammonia weight ratio not exceeding 5:1 . This ensures the formation of monothis compound while minimizing the production of dithis compound and nitrogen trichloride.
Chemical Reactions Analysis
Types of Reactions: Chloramine undergoes various chemical reactions, including:
Oxidation: this compound can be oxidized to form dithis compound and nitrogen trichloride under certain conditions.
Reduction: this compound can be reduced back to ammonia and chlorine.
Substitution: this compound can participate in substitution reactions, where the chlorine atom is replaced by other substituents.
Common Reagents and Conditions:
Oxidation: Acidic conditions favor the oxidation of monothis compound to dithis compound and nitrogen trichloride.
Substitution: this compound reacts with secondary amines in the presence of sodium hypochlorite to form substituted chloramines.
Major Products:
- Dithis compound (NHCl2)
- Nitrogen trichloride (NCl3)
- Substituted chloramines
Scientific Research Applications
Chloramine has a wide range of applications in scientific research, including:
- Chemistry: Used as a reagent in organic synthesis and analytical chemistry .
- Biology: Employed in the disinfection of laboratory equipment and biological samples .
- Medicine: Utilized as an antiseptic and disinfectant in healthcare settings .
- Industry: Applied in water treatment to ensure microbiological safety in drinking water distribution systems .
Mechanism of Action
Chloramine exerts its disinfectant effects by penetrating the cell walls of microorganisms and disrupting their metabolic processes. The chlorine component of this compound reacts with the proteins and enzymes within the cells, leading to their inactivation and death . This mechanism ensures prolonged disinfection as this compound remains stable in water for extended periods.
Comparison with Similar Compounds
- Chlorine (Cl2): A more reactive disinfectant that provides immediate disinfection but does not last as long as chloramine in water .
- Bromamine: Similar to this compound but contains bromine instead of chlorine.
- Iodamine: Contains iodine and is used in specific disinfection applications.
Uniqueness of this compound: this compound is unique in its ability to provide long-lasting disinfection while producing fewer disinfection by-products compared to chlorine . This makes it particularly suitable for water treatment in distribution systems with long detention times.
Properties
InChI |
InChI=1S/ClH2N/c1-2/h2H2 | |
---|---|---|
Source | PubChem | |
URL | https://pubchem.ncbi.nlm.nih.gov | |
Description | Data deposited in or computed by PubChem | |
InChI Key |
QDHHCQZDFGDHMP-UHFFFAOYSA-N | |
Source | PubChem | |
URL | https://pubchem.ncbi.nlm.nih.gov | |
Description | Data deposited in or computed by PubChem | |
Canonical SMILES |
NCl | |
Source | PubChem | |
URL | https://pubchem.ncbi.nlm.nih.gov | |
Description | Data deposited in or computed by PubChem | |
Molecular Formula |
ClH2N, NH2Cl | |
Record name | CHLORAMINE | |
Source | CAMEO Chemicals | |
URL | https://cameochemicals.noaa.gov/chemical/19968 | |
Description | CAMEO Chemicals is a chemical database designed for people who are involved in hazardous material incident response and planning. CAMEO Chemicals contains a library with thousands of datasheets containing response-related information and recommendations for hazardous materials that are commonly transported, used, or stored in the United States. CAMEO Chemicals was developed by the National Oceanic and Atmospheric Administration's Office of Response and Restoration in partnership with the Environmental Protection Agency's Office of Emergency Management. | |
Explanation | CAMEO Chemicals and all other CAMEO products are available at no charge to those organizations and individuals (recipients) responsible for the safe handling of chemicals. However, some of the chemical data itself is subject to the copyright restrictions of the companies or organizations that provided the data. | |
Record name | Chloramine | |
Source | Wikipedia | |
URL | https://en.wikipedia.org/wiki/Chloramine | |
Description | Chemical information link to Wikipedia. | |
Source | PubChem | |
URL | https://pubchem.ncbi.nlm.nih.gov | |
Description | Data deposited in or computed by PubChem | |
DSSTOX Substance ID |
DTXSID8023842, DTXSID00721369 | |
Record name | Chloramine | |
Source | EPA DSSTox | |
URL | https://comptox.epa.gov/dashboard/DTXSID8023842 | |
Description | DSSTox provides a high quality public chemistry resource for supporting improved predictive toxicology. | |
Record name | PUBCHEM_57347134 | |
Source | EPA DSSTox | |
URL | https://comptox.epa.gov/dashboard/DTXSID00721369 | |
Description | DSSTox provides a high quality public chemistry resource for supporting improved predictive toxicology. | |
Molecular Weight |
51.47 g/mol | |
Source | PubChem | |
URL | https://pubchem.ncbi.nlm.nih.gov | |
Description | Data deposited in or computed by PubChem | |
Physical Description |
Chloramine is a colorless to yellow liquid with a strong pungent odor. (NTP, 1992) | |
Record name | CHLORAMINE | |
Source | CAMEO Chemicals | |
URL | https://cameochemicals.noaa.gov/chemical/19968 | |
Description | CAMEO Chemicals is a chemical database designed for people who are involved in hazardous material incident response and planning. CAMEO Chemicals contains a library with thousands of datasheets containing response-related information and recommendations for hazardous materials that are commonly transported, used, or stored in the United States. CAMEO Chemicals was developed by the National Oceanic and Atmospheric Administration's Office of Response and Restoration in partnership with the Environmental Protection Agency's Office of Emergency Management. | |
Explanation | CAMEO Chemicals and all other CAMEO products are available at no charge to those organizations and individuals (recipients) responsible for the safe handling of chemicals. However, some of the chemical data itself is subject to the copyright restrictions of the companies or organizations that provided the data. | |
Solubility |
Soluble (NTP, 1992), Soluble in water, alcohol and ether, SOL IN COLD WATER, ETHER; VERY SLIGHTLY SOL IN CARBON TETRACHLORIDE, BENZENE | |
Record name | CHLORAMINE | |
Source | CAMEO Chemicals | |
URL | https://cameochemicals.noaa.gov/chemical/19968 | |
Description | CAMEO Chemicals is a chemical database designed for people who are involved in hazardous material incident response and planning. CAMEO Chemicals contains a library with thousands of datasheets containing response-related information and recommendations for hazardous materials that are commonly transported, used, or stored in the United States. CAMEO Chemicals was developed by the National Oceanic and Atmospheric Administration's Office of Response and Restoration in partnership with the Environmental Protection Agency's Office of Emergency Management. | |
Explanation | CAMEO Chemicals and all other CAMEO products are available at no charge to those organizations and individuals (recipients) responsible for the safe handling of chemicals. However, some of the chemical data itself is subject to the copyright restrictions of the companies or organizations that provided the data. | |
Record name | CHLORAMINE | |
Source | Hazardous Substances Data Bank (HSDB) | |
URL | https://pubchem.ncbi.nlm.nih.gov/source/hsdb/4293 | |
Description | The Hazardous Substances Data Bank (HSDB) is a toxicology database that focuses on the toxicology of potentially hazardous chemicals. It provides information on human exposure, industrial hygiene, emergency handling procedures, environmental fate, regulatory requirements, nanomaterials, and related areas. The information in HSDB has been assessed by a Scientific Review Panel. | |
Mechanism of Action |
On dissolution of the chloramines in the epithelial lining fluid, hypochlorous acid, ammonia, and oxygen-radicals are generated, all of which act as irritants. /Chloramines/ | |
Record name | CHLORAMINE | |
Source | Hazardous Substances Data Bank (HSDB) | |
URL | https://pubchem.ncbi.nlm.nih.gov/source/hsdb/4293 | |
Description | The Hazardous Substances Data Bank (HSDB) is a toxicology database that focuses on the toxicology of potentially hazardous chemicals. It provides information on human exposure, industrial hygiene, emergency handling procedures, environmental fate, regulatory requirements, nanomaterials, and related areas. The information in HSDB has been assessed by a Scientific Review Panel. | |
Color/Form |
YELLOW LIQUID, Colorless liquid | |
CAS No. |
10599-90-3, 12190-75-9 | |
Record name | CHLORAMINE | |
Source | CAMEO Chemicals | |
URL | https://cameochemicals.noaa.gov/chemical/19968 | |
Description | CAMEO Chemicals is a chemical database designed for people who are involved in hazardous material incident response and planning. CAMEO Chemicals contains a library with thousands of datasheets containing response-related information and recommendations for hazardous materials that are commonly transported, used, or stored in the United States. CAMEO Chemicals was developed by the National Oceanic and Atmospheric Administration's Office of Response and Restoration in partnership with the Environmental Protection Agency's Office of Emergency Management. | |
Explanation | CAMEO Chemicals and all other CAMEO products are available at no charge to those organizations and individuals (recipients) responsible for the safe handling of chemicals. However, some of the chemical data itself is subject to the copyright restrictions of the companies or organizations that provided the data. | |
Record name | Monochloramine | |
Source | CAS Common Chemistry | |
URL | https://commonchemistry.cas.org/detail?cas_rn=10599-90-3 | |
Description | CAS Common Chemistry is an open community resource for accessing chemical information. Nearly 500,000 chemical substances from CAS REGISTRY cover areas of community interest, including common and frequently regulated chemicals, and those relevant to high school and undergraduate chemistry classes. This chemical information, curated by our expert scientists, is provided in alignment with our mission as a division of the American Chemical Society. | |
Explanation | The data from CAS Common Chemistry is provided under a CC-BY-NC 4.0 license, unless otherwise stated. | |
Record name | Chloramine | |
Source | ChemIDplus | |
URL | https://pubchem.ncbi.nlm.nih.gov/substance/?source=chemidplus&sourceid=0010599903 | |
Description | ChemIDplus is a free, web search system that provides access to the structure and nomenclature authority files used for the identification of chemical substances cited in National Library of Medicine (NLM) databases, including the TOXNET system. | |
Record name | Chloramine | |
Source | EPA DSSTox | |
URL | https://comptox.epa.gov/dashboard/DTXSID8023842 | |
Description | DSSTox provides a high quality public chemistry resource for supporting improved predictive toxicology. | |
Record name | PUBCHEM_57347134 | |
Source | EPA DSSTox | |
URL | https://comptox.epa.gov/dashboard/DTXSID00721369 | |
Description | DSSTox provides a high quality public chemistry resource for supporting improved predictive toxicology. | |
Record name | CHLORAMINE | |
Source | FDA Global Substance Registration System (GSRS) | |
URL | https://gsrs.ncats.nih.gov/ginas/app/beta/substances/KW8K411A1P | |
Description | The FDA Global Substance Registration System (GSRS) enables the efficient and accurate exchange of information on what substances are in regulated products. Instead of relying on names, which vary across regulatory domains, countries, and regions, the GSRS knowledge base makes it possible for substances to be defined by standardized, scientific descriptions. | |
Explanation | Unless otherwise noted, the contents of the FDA website (www.fda.gov), both text and graphics, are not copyrighted. They are in the public domain and may be republished, reprinted and otherwise used freely by anyone without the need to obtain permission from FDA. Credit to the U.S. Food and Drug Administration as the source is appreciated but not required. | |
Record name | CHLORAMINE | |
Source | Hazardous Substances Data Bank (HSDB) | |
URL | https://pubchem.ncbi.nlm.nih.gov/source/hsdb/4293 | |
Description | The Hazardous Substances Data Bank (HSDB) is a toxicology database that focuses on the toxicology of potentially hazardous chemicals. It provides information on human exposure, industrial hygiene, emergency handling procedures, environmental fate, regulatory requirements, nanomaterials, and related areas. The information in HSDB has been assessed by a Scientific Review Panel. | |
Melting Point |
-87 °F (NTP, 1992), -66 °C | |
Record name | CHLORAMINE | |
Source | CAMEO Chemicals | |
URL | https://cameochemicals.noaa.gov/chemical/19968 | |
Description | CAMEO Chemicals is a chemical database designed for people who are involved in hazardous material incident response and planning. CAMEO Chemicals contains a library with thousands of datasheets containing response-related information and recommendations for hazardous materials that are commonly transported, used, or stored in the United States. CAMEO Chemicals was developed by the National Oceanic and Atmospheric Administration's Office of Response and Restoration in partnership with the Environmental Protection Agency's Office of Emergency Management. | |
Explanation | CAMEO Chemicals and all other CAMEO products are available at no charge to those organizations and individuals (recipients) responsible for the safe handling of chemicals. However, some of the chemical data itself is subject to the copyright restrictions of the companies or organizations that provided the data. | |
Record name | CHLORAMINE | |
Source | Hazardous Substances Data Bank (HSDB) | |
URL | https://pubchem.ncbi.nlm.nih.gov/source/hsdb/4293 | |
Description | The Hazardous Substances Data Bank (HSDB) is a toxicology database that focuses on the toxicology of potentially hazardous chemicals. It provides information on human exposure, industrial hygiene, emergency handling procedures, environmental fate, regulatory requirements, nanomaterials, and related areas. The information in HSDB has been assessed by a Scientific Review Panel. | |
Retrosynthesis Analysis
AI-Powered Synthesis Planning: Our tool employs the Template_relevance Pistachio, Template_relevance Bkms_metabolic, Template_relevance Pistachio_ringbreaker, Template_relevance Reaxys, Template_relevance Reaxys_biocatalysis model, leveraging a vast database of chemical reactions to predict feasible synthetic routes.
One-Step Synthesis Focus: Specifically designed for one-step synthesis, it provides concise and direct routes for your target compounds, streamlining the synthesis process.
Accurate Predictions: Utilizing the extensive PISTACHIO, BKMS_METABOLIC, PISTACHIO_RINGBREAKER, REAXYS, REAXYS_BIOCATALYSIS database, our tool offers high-accuracy predictions, reflecting the latest in chemical research and data.
Strategy Settings
Precursor scoring | Relevance Heuristic |
---|---|
Min. plausibility | 0.01 |
Model | Template_relevance |
Template Set | Pistachio/Bkms_metabolic/Pistachio_ringbreaker/Reaxys/Reaxys_biocatalysis |
Top-N result to add to graph | 6 |
Feasible Synthetic Routes
Disclaimer and Information on In-Vitro Research Products
Please be aware that all articles and product information presented on BenchChem are intended solely for informational purposes. The products available for purchase on BenchChem are specifically designed for in-vitro studies, which are conducted outside of living organisms. In-vitro studies, derived from the Latin term "in glass," involve experiments performed in controlled laboratory settings using cells or tissues. It is important to note that these products are not categorized as medicines or drugs, and they have not received approval from the FDA for the prevention, treatment, or cure of any medical condition, ailment, or disease. We must emphasize that any form of bodily introduction of these products into humans or animals is strictly prohibited by law. It is essential to adhere to these guidelines to ensure compliance with legal and ethical standards in research and experimentation.