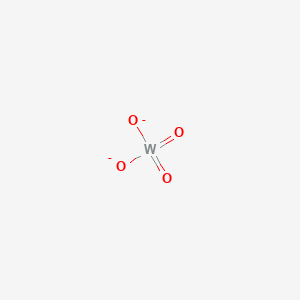
Tungstate
Overview
Description
Tungstate is a compound that contains an oxyanion of tungsten or is a mixed oxide containing tungsten . The simplest this compound ion is WO2− 4, "orthothis compound" . Many other tungstates belong to a large group of polyatomic ions that are termed polyoxometalates .
Synthesis Analysis
Bismuth this compound photocatalysts with different morphologies were synthesized by simple hydrothermal synthesis under different pH and different hydrothermal reaction time . Copper this compound nanoparticles were prepared by Solid state synthesis .
Molecular Structure Analysis
Orthotungstates feature tetrahedral W (VI) centres with short W–O distances of 1.79 Å . Structurally, they resemble sulfates . Six-coordinate, octahedral tungsten dominates in the polyoxotungstates .
Chemical Reactions Analysis
Solutions of tungstates give intensely blue solutions of complex this compound (V,VI) analogous to the molybdenum blues when reduced by most organic materials . Unlike chromate, this compound is not a good oxidizer, but like chromate, solutions of this compound condense to give the isopolytungstates upon acidification .
Physical And Chemical Properties Analysis
Tungsten has an atomic number of 74, a relative atomic mass of 183.85, and an atomic volume of 9.53 cm3 / mol . Tungsten is stable to mineral acids in the cold, and is only slightly attacked at higher temperatures .
Scientific Research Applications
Scintillator Development : Lead tungstate is used in large, fundamental research projects to enhance detector performances for ambitious scientific goals. It's also applied in commercial systems like security and medical imaging devices (Lecoq, Gektin, & Korzhik, 2017).
Laser Technology : this compound-doped crystals are used in lasers, particularly in the 2 µm spectral range, relevant for medicine, environmental gas detection, and scientific research (Bolschikov et al., 2011).
Raman Lasers : this compound crystals, like barium this compound and calcium molybdate, are used in Raman lasers due to their thermal and physical properties, high moisture resistance, and efficiency in new spectral regions (Ivleva et al., 2013).
Antidiabetic Research : this compound shows potential as an oral antidiabetic agent in animal models of diabetes, though concerns about chronic toxicity and tissue accumulation exist (Domingo, 2002).
Plant Physiology : this compound affects nitrate assimilation in higher plant tissues and can be used to study the regulation of nitrate uptake and other steps in the nitrate assimilation pathway in plants (Heimer, Wray, & Filner, 1969).
Purification of Sodium this compound : Important for producing high-quality laser crystals, the purification process of sodium this compound is critical for the performance of neodymium-doped calcium this compound crystals used in lasers (Gates & Cockayne, 1965).
This compound Toxicity and Environmental Impact : Studies on the acute toxicity of tungstates to fish show that sodium metathis compound is significantly more toxic than sodium this compound, indicating varying environmental effects based on tungsten speciation (Strigul, Koutsospyros, & Christodoulatos, 2010).
Corrosion Inhibition : Sodium this compound, in combination with potassium iodate, is used as a corrosion inhibitor in industrial cooling water systems. Its co-inhibition characteristics are significant in the formation and strength of the passive film on mild steel (Shibli & Saji, 2005).
Nanotechnology : this compound nanoparticles, including lead, cadmium, calcium, and zinc tungstates, are synthesized for use as scintillators in detector materials for high-energy radiation. The sol-gel method allows production at room temperatures, offering advantages in manufacturing (Nadaraia et al., 2010).
Two-Dimensional Tungstates : Copper this compound (CuWO4) has been fabricated as a two-dimensional material, showing promise for applications in photo- and electrocatalysis due to its unique structural and electronic properties (Denk et al., 2014).
Mechanism of Action
Safety and Hazards
Future Directions
Bismuth tungstate (Bi2WO6) has received extensive research in a numerous area, including degradation, CO2 reduction, organic transformations, etc . Due to their wide range of applications, the discovery and development of effective, environmentally safe, gentle, and affordable techniques for the synthesis of bismuth this compound are critical .
properties
IUPAC Name |
dioxido(dioxo)tungsten | |
---|---|---|
Source | PubChem | |
URL | https://pubchem.ncbi.nlm.nih.gov | |
Description | Data deposited in or computed by PubChem | |
InChI |
InChI=1S/4O.W/q;;2*-1; | |
Source | PubChem | |
URL | https://pubchem.ncbi.nlm.nih.gov | |
Description | Data deposited in or computed by PubChem | |
InChI Key |
PBYZMCDFOULPGH-UHFFFAOYSA-N | |
Source | PubChem | |
URL | https://pubchem.ncbi.nlm.nih.gov | |
Description | Data deposited in or computed by PubChem | |
Canonical SMILES |
[O-][W](=O)(=O)[O-] | |
Source | PubChem | |
URL | https://pubchem.ncbi.nlm.nih.gov | |
Description | Data deposited in or computed by PubChem | |
Molecular Formula |
O4W-2 | |
Source | PubChem | |
URL | https://pubchem.ncbi.nlm.nih.gov | |
Description | Data deposited in or computed by PubChem | |
Related CAS |
51682-10-1 (Parent), Array | |
Record name | Tungstate ion | |
Source | ChemIDplus | |
URL | https://pubchem.ncbi.nlm.nih.gov/substance/?source=chemidplus&sourceid=0012737869 | |
Description | ChemIDplus is a free, web search system that provides access to the structure and nomenclature authority files used for the identification of chemical substances cited in National Library of Medicine (NLM) databases, including the TOXNET system. | |
Record name | Tungstate | |
Source | ChemIDplus | |
URL | https://pubchem.ncbi.nlm.nih.gov/substance/?source=chemidplus&sourceid=0051682101 | |
Description | ChemIDplus is a free, web search system that provides access to the structure and nomenclature authority files used for the identification of chemical substances cited in National Library of Medicine (NLM) databases, including the TOXNET system. | |
Molecular Weight |
247.84 g/mol | |
Source | PubChem | |
URL | https://pubchem.ncbi.nlm.nih.gov | |
Description | Data deposited in or computed by PubChem | |
CAS RN |
12737-86-9, 51682-10-1 | |
Record name | Tungstate ion | |
Source | ChemIDplus | |
URL | https://pubchem.ncbi.nlm.nih.gov/substance/?source=chemidplus&sourceid=0012737869 | |
Description | ChemIDplus is a free, web search system that provides access to the structure and nomenclature authority files used for the identification of chemical substances cited in National Library of Medicine (NLM) databases, including the TOXNET system. | |
Record name | Tungstate | |
Source | ChemIDplus | |
URL | https://pubchem.ncbi.nlm.nih.gov/substance/?source=chemidplus&sourceid=0051682101 | |
Description | ChemIDplus is a free, web search system that provides access to the structure and nomenclature authority files used for the identification of chemical substances cited in National Library of Medicine (NLM) databases, including the TOXNET system. | |
Record name | TUNGSTATE ION | |
Source | FDA Global Substance Registration System (GSRS) | |
URL | https://gsrs.ncats.nih.gov/ginas/app/beta/substances/SW0Y0WQ46I | |
Description | The FDA Global Substance Registration System (GSRS) enables the efficient and accurate exchange of information on what substances are in regulated products. Instead of relying on names, which vary across regulatory domains, countries, and regions, the GSRS knowledge base makes it possible for substances to be defined by standardized, scientific descriptions. | |
Explanation | Unless otherwise noted, the contents of the FDA website (www.fda.gov), both text and graphics, are not copyrighted. They are in the public domain and may be republished, reprinted and otherwise used freely by anyone without the need to obtain permission from FDA. Credit to the U.S. Food and Drug Administration as the source is appreciated but not required. | |
Disclaimer and Information on In-Vitro Research Products
Please be aware that all articles and product information presented on BenchChem are intended solely for informational purposes. The products available for purchase on BenchChem are specifically designed for in-vitro studies, which are conducted outside of living organisms. In-vitro studies, derived from the Latin term "in glass," involve experiments performed in controlled laboratory settings using cells or tissues. It is important to note that these products are not categorized as medicines or drugs, and they have not received approval from the FDA for the prevention, treatment, or cure of any medical condition, ailment, or disease. We must emphasize that any form of bodily introduction of these products into humans or animals is strictly prohibited by law. It is essential to adhere to these guidelines to ensure compliance with legal and ethical standards in research and experimentation.