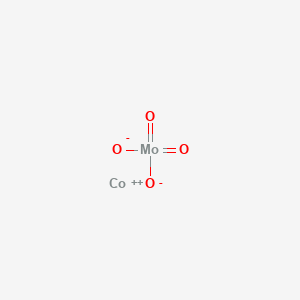
Cobalt molybdate
Overview
Description
Cobalt molybdate is an inorganic compound with the chemical formula CoMoO₄. It is known for its unique properties and applications in various fields, including catalysis, electronics, and materials science. This compound exists in several phases, including the low-temperature alpha-phase, high-temperature beta-phase, high-pressure phase, and hydrate forms. This compound is particularly noted for its catalytic activity in oxidation reactions and its potential in photocatalytic applications .
Preparation Methods
Synthetic Routes and Reaction Conditions: Cobalt molybdate can be synthesized through various methods, including:
Sonochemical Method: This involves the reaction between cobalt(II) nitrate hexahydrate and ammonium heptamolybdate tetrahydrate in water.
Solid-State Reaction: This method involves the reaction of molybdenum trioxide with cobalt compounds at high temperatures, typically around 1000°C.
Hydrothermal Method: This involves the reaction of cobalt chloride and ammonium molybdate in the presence of urea as a precipitation agent and structure control agent.
Industrial Production Methods: Industrial production of this compound often involves large-scale solid-state reactions or hydrothermal methods due to their scalability and efficiency. These methods ensure the production of this compound with high purity and desired morphological characteristics.
Chemical Reactions Analysis
Cobalt molybdate undergoes various chemical reactions, including:
Oxidation Reactions: It acts as a catalyst in the oxidation of hydrocarbons.
Reduction Reactions: this compound can be reduced to form cobalt and molybdenum oxides under specific conditions.
Substitution Reactions: It can undergo substitution reactions where cobalt or molybdenum atoms are replaced by other metal atoms, leading to the formation of doped or substituted molybdates.
Common Reagents and Conditions:
Oxidation: Hydrocarbons and oxygen are common reagents, with reactions typically occurring at elevated temperatures.
Reduction: Hydrogen or other reducing agents are used under controlled conditions.
Substitution: Metal salts are used in aqueous or solid-state reactions.
Major Products:
Oxidation: Oxidized hydrocarbons and reoxidized this compound.
Reduction: Cobalt and molybdenum oxides.
Substitution: Doped or substituted molybdates with altered properties.
Scientific Research Applications
Cobalt molybdate has a wide range of scientific research applications, including:
Catalysis: It is used as a catalyst in the oxidation of hydrocarbons and other organic compounds.
Photocatalysis: It is employed in the photocatalytic degradation of organic pollutants under ultraviolet light.
Electrocatalysis: this compound is used in electrocatalysts for hydrogen evolution reactions and oxygen evolution reactions.
Energy Storage: It is utilized in supercapacitors and rechargeable batteries due to its excellent electrochemical properties.
Sensors: this compound is used in humidity sensors and other types of chemical sensors.
Mechanism of Action
The mechanism of action of cobalt molybdate in catalytic processes involves the redox mechanism. In oxidation reactions, the hydrocarbon molecule is oxidized by an oxygen ion from the this compound lattice. The catalyst is then reoxidized by oxygen from the gas phase, completing the catalytic cycle . In electrocatalysis, this compound facilitates the transfer of electrons, enhancing the efficiency of hydrogen and oxygen evolution reactions .
Comparison with Similar Compounds
Cobalt molybdate can be compared with other similar compounds, such as:
Nickel Molybdate (NiMoO₄): Similar to this compound, nickel molybdate is used in catalysis and energy storage applications.
Copper Molybdate (CuMoO₄): Copper molybdate is another transition metal molybdate with applications in catalysis and materials science.
Tungsten-Doped this compound (W-CoMoO₄): Doping this compound with tungsten enhances its catalytic properties and stability, making it a superior catalyst for certain reactions.
This compound stands out due to its unique combination of catalytic activity, stability, and versatility in various applications.
Properties
IUPAC Name |
cobalt(2+);dioxido(dioxo)molybdenum | |
---|---|---|
Source | PubChem | |
URL | https://pubchem.ncbi.nlm.nih.gov | |
Description | Data deposited in or computed by PubChem | |
InChI |
InChI=1S/Co.Mo.4O/q+2;;;;2*-1 | |
Source | PubChem | |
URL | https://pubchem.ncbi.nlm.nih.gov | |
Description | Data deposited in or computed by PubChem | |
InChI Key |
KYYSIVCCYWZZLR-UHFFFAOYSA-N | |
Source | PubChem | |
URL | https://pubchem.ncbi.nlm.nih.gov | |
Description | Data deposited in or computed by PubChem | |
Canonical SMILES |
[O-][Mo](=O)(=O)[O-].[Co+2] | |
Source | PubChem | |
URL | https://pubchem.ncbi.nlm.nih.gov | |
Description | Data deposited in or computed by PubChem | |
Molecular Formula |
CoMoO4 | |
Record name | cobalt(II) molybdate | |
Source | Wikipedia | |
URL | https://en.wikipedia.org/wiki/Dictionary_of_chemical_formulas | |
Description | Chemical information link to Wikipedia. | |
Source | PubChem | |
URL | https://pubchem.ncbi.nlm.nih.gov | |
Description | Data deposited in or computed by PubChem | |
Molecular Weight |
218.88 g/mol | |
Source | PubChem | |
URL | https://pubchem.ncbi.nlm.nih.gov | |
Description | Data deposited in or computed by PubChem | |
Physical Description |
Liquid; Other Solid; Pellets or Large Crystals, Gray-green solid; [Hawley] Green powder, insoluble in water; [MSDSonline] | |
Record name | Cobalt molybdenum oxide (CoMoO4) | |
Source | EPA Chemicals under the TSCA | |
URL | https://www.epa.gov/chemicals-under-tsca | |
Description | EPA Chemicals under the Toxic Substances Control Act (TSCA) collection contains information on chemicals and their regulations under TSCA, including non-confidential content from the TSCA Chemical Substance Inventory and Chemical Data Reporting. | |
Record name | Cobalt(II) molybdate | |
Source | Haz-Map, Information on Hazardous Chemicals and Occupational Diseases | |
URL | https://haz-map.com/Agents/8035 | |
Description | Haz-Map® is an occupational health database designed for health and safety professionals and for consumers seeking information about the adverse effects of workplace exposures to chemical and biological agents. | |
Explanation | Copyright (c) 2022 Haz-Map(R). All rights reserved. Unless otherwise indicated, all materials from Haz-Map are copyrighted by Haz-Map(R). No part of these materials, either text or image may be used for any purpose other than for personal use. Therefore, reproduction, modification, storage in a retrieval system or retransmission, in any form or by any means, electronic, mechanical or otherwise, for reasons other than personal use, is strictly prohibited without prior written permission. | |
CAS No. |
13762-14-6 | |
Record name | Cobalt molybdenum oxide (CoMoO4) | |
Source | ChemIDplus | |
URL | https://pubchem.ncbi.nlm.nih.gov/substance/?source=chemidplus&sourceid=0013762146 | |
Description | ChemIDplus is a free, web search system that provides access to the structure and nomenclature authority files used for the identification of chemical substances cited in National Library of Medicine (NLM) databases, including the TOXNET system. | |
Record name | Cobalt molybdenum oxide (CoMoO4) | |
Source | EPA Chemicals under the TSCA | |
URL | https://www.epa.gov/chemicals-under-tsca | |
Description | EPA Chemicals under the Toxic Substances Control Act (TSCA) collection contains information on chemicals and their regulations under TSCA, including non-confidential content from the TSCA Chemical Substance Inventory and Chemical Data Reporting. | |
Record name | Cobalt molybdate | |
Source | European Chemicals Agency (ECHA) | |
URL | https://echa.europa.eu/substance-information/-/substanceinfo/100.033.948 | |
Description | The European Chemicals Agency (ECHA) is an agency of the European Union which is the driving force among regulatory authorities in implementing the EU's groundbreaking chemicals legislation for the benefit of human health and the environment as well as for innovation and competitiveness. | |
Explanation | Use of the information, documents and data from the ECHA website is subject to the terms and conditions of this Legal Notice, and subject to other binding limitations provided for under applicable law, the information, documents and data made available on the ECHA website may be reproduced, distributed and/or used, totally or in part, for non-commercial purposes provided that ECHA is acknowledged as the source: "Source: European Chemicals Agency, http://echa.europa.eu/". Such acknowledgement must be included in each copy of the material. ECHA permits and encourages organisations and individuals to create links to the ECHA website under the following cumulative conditions: Links can only be made to webpages that provide a link to the Legal Notice page. | |
Disclaimer and Information on In-Vitro Research Products
Please be aware that all articles and product information presented on BenchChem are intended solely for informational purposes. The products available for purchase on BenchChem are specifically designed for in-vitro studies, which are conducted outside of living organisms. In-vitro studies, derived from the Latin term "in glass," involve experiments performed in controlled laboratory settings using cells or tissues. It is important to note that these products are not categorized as medicines or drugs, and they have not received approval from the FDA for the prevention, treatment, or cure of any medical condition, ailment, or disease. We must emphasize that any form of bodily introduction of these products into humans or animals is strictly prohibited by law. It is essential to adhere to these guidelines to ensure compliance with legal and ethical standards in research and experimentation.