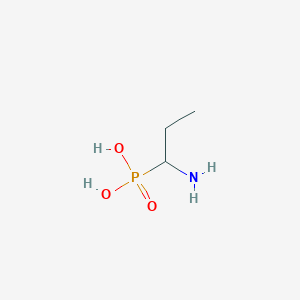
1-Aminopropylphosphonic acid
Overview
Description
1-Aminopropylphosphonic acid is a useful research compound. Its molecular formula is C3H10NO3P and its molecular weight is 139.09 g/mol. The purity is usually 95%.
The exact mass of the compound this compound is unknown and the complexity rating of the compound is unknown. The compound has been submitted to the National Cancer Institute (NCI) for testing and evaluation and the Cancer Chemotherapy National Service Center (NSC) number is 133879. The United Nations designated GHS hazard class pictogram is Corrosive, and the GHS signal word is DangerThe storage condition is unknown. Please store according to label instructions upon receipt of goods.Use and application categories indicated by third-party sources: Agrochemicals -> Fungicides. However, this does not mean our product can be used or applied in the same or a similar way.
BenchChem offers high-quality this compound suitable for many research applications. Different packaging options are available to accommodate customers' requirements. Please inquire for more information about this compound including the price, delivery time, and more detailed information at info@benchchem.com.
Biological Activity
1-Aminopropylphosphonic acid (1-APPA) is a compound of significant interest in medicinal chemistry and biochemistry due to its diverse biological activities. This article explores its biological mechanisms, pharmacological effects, and potential therapeutic applications based on recent research findings.
Chemical Structure and Properties
This compound is characterized by the presence of a phosphonic acid group attached to an aminopropyl chain. Its molecular formula is C₃H₉NO₃P, and it has a molecular weight of approximately 153.08 g/mol. The compound exhibits both acidic and basic properties, making it versatile in various biochemical interactions.
1-APPA's biological activity is primarily attributed to its ability to interact with various enzymes and receptors. Notably, it acts as an inhibitor of certain enzymes involved in amino acid metabolism.
Enzyme Inhibition
Research indicates that 1-APPA inhibits alanine racemase, an enzyme crucial for the interconversion of L-alanine and D-alanine. This inhibition is significant because D-alanine is essential for bacterial cell wall synthesis, suggesting that 1-APPA could have antibacterial properties . The kinetic characterization of this interaction reveals that 1-APPA has a higher affinity for the enzyme compared to other phosphonic acid derivatives, which enhances its potential as an antimicrobial agent.
Biological Activities
The biological activities of 1-APPA can be categorized into several key areas:
- Antimicrobial Activity : Studies have demonstrated that 1-APPA exhibits antibacterial effects against various Gram-positive and Gram-negative bacteria. Its mechanism involves the disruption of bacterial cell wall synthesis due to alanine racemase inhibition .
- Neuroprotective Effects : There is emerging evidence suggesting that 1-APPA may have neuroprotective properties. It has been shown to modulate neurotransmitter levels, particularly GABA, which could be beneficial in treating neurological disorders .
- Anticancer Potential : Preliminary studies indicate that 1-APPA may inhibit the proliferation of certain cancer cell lines by inducing apoptosis. The exact mechanisms are still under investigation but may involve the modulation of signaling pathways related to cell survival and death .
Case Studies and Experimental Findings
Several studies have explored the biological activity of 1-APPA in various experimental settings:
Table 1: Summary of Biological Activities
Activity Type | Observed Effects | References |
---|---|---|
Antimicrobial | Inhibits growth of Gram-positive bacteria | |
Neuroprotective | Modulates GABA levels | |
Anticancer | Induces apoptosis in cancer cells |
Table 2: Kinetic Parameters for Enzyme Inhibition
Properties
IUPAC Name |
1-aminopropylphosphonic acid | |
---|---|---|
Source | PubChem | |
URL | https://pubchem.ncbi.nlm.nih.gov | |
Description | Data deposited in or computed by PubChem | |
InChI |
InChI=1S/C3H10NO3P/c1-2-3(4)8(5,6)7/h3H,2,4H2,1H3,(H2,5,6,7) | |
Source | PubChem | |
URL | https://pubchem.ncbi.nlm.nih.gov | |
Description | Data deposited in or computed by PubChem | |
InChI Key |
DELJNDWGTWHHFA-UHFFFAOYSA-N | |
Source | PubChem | |
URL | https://pubchem.ncbi.nlm.nih.gov | |
Description | Data deposited in or computed by PubChem | |
Canonical SMILES |
CCC(N)P(=O)(O)O | |
Source | PubChem | |
URL | https://pubchem.ncbi.nlm.nih.gov | |
Description | Data deposited in or computed by PubChem | |
Molecular Formula |
C3H10NO3P | |
Source | PubChem | |
URL | https://pubchem.ncbi.nlm.nih.gov | |
Description | Data deposited in or computed by PubChem | |
DSSTOX Substance ID |
DTXSID50864694 | |
Record name | Ampropylfos | |
Source | EPA DSSTox | |
URL | https://comptox.epa.gov/dashboard/DTXSID50864694 | |
Description | DSSTox provides a high quality public chemistry resource for supporting improved predictive toxicology. | |
Molecular Weight |
139.09 g/mol | |
Source | PubChem | |
URL | https://pubchem.ncbi.nlm.nih.gov | |
Description | Data deposited in or computed by PubChem | |
CAS No. |
14047-23-5 | |
Record name | Ampropylfos [INN] | |
Source | ChemIDplus | |
URL | https://pubchem.ncbi.nlm.nih.gov/substance/?source=chemidplus&sourceid=0014047235 | |
Description | ChemIDplus is a free, web search system that provides access to the structure and nomenclature authority files used for the identification of chemical substances cited in National Library of Medicine (NLM) databases, including the TOXNET system. | |
Record name | NSC-133879 | |
Source | DTP/NCI | |
URL | https://dtp.cancer.gov/dtpstandard/servlet/dwindex?searchtype=NSC&outputformat=html&searchlist=133879 | |
Description | The NCI Development Therapeutics Program (DTP) provides services and resources to the academic and private-sector research communities worldwide to facilitate the discovery and development of new cancer therapeutic agents. | |
Explanation | Unless otherwise indicated, all text within NCI products is free of copyright and may be reused without our permission. Credit the National Cancer Institute as the source. | |
Record name | Ampropylfos | |
Source | EPA DSSTox | |
URL | https://comptox.epa.gov/dashboard/DTXSID50864694 | |
Description | DSSTox provides a high quality public chemistry resource for supporting improved predictive toxicology. | |
Record name | Phosphonic acid, (1-aminopropyl)- | |
Source | European Chemicals Agency (ECHA) | |
URL | https://echa.europa.eu/information-on-chemicals | |
Description | The European Chemicals Agency (ECHA) is an agency of the European Union which is the driving force among regulatory authorities in implementing the EU's groundbreaking chemicals legislation for the benefit of human health and the environment as well as for innovation and competitiveness. | |
Explanation | Use of the information, documents and data from the ECHA website is subject to the terms and conditions of this Legal Notice, and subject to other binding limitations provided for under applicable law, the information, documents and data made available on the ECHA website may be reproduced, distributed and/or used, totally or in part, for non-commercial purposes provided that ECHA is acknowledged as the source: "Source: European Chemicals Agency, http://echa.europa.eu/". Such acknowledgement must be included in each copy of the material. ECHA permits and encourages organisations and individuals to create links to the ECHA website under the following cumulative conditions: Links can only be made to webpages that provide a link to the Legal Notice page. | |
Record name | AMPROPYLFOS | |
Source | FDA Global Substance Registration System (GSRS) | |
URL | https://gsrs.ncats.nih.gov/ginas/app/beta/substances/60BDS7L11G | |
Description | The FDA Global Substance Registration System (GSRS) enables the efficient and accurate exchange of information on what substances are in regulated products. Instead of relying on names, which vary across regulatory domains, countries, and regions, the GSRS knowledge base makes it possible for substances to be defined by standardized, scientific descriptions. | |
Explanation | Unless otherwise noted, the contents of the FDA website (www.fda.gov), both text and graphics, are not copyrighted. They are in the public domain and may be republished, reprinted and otherwise used freely by anyone without the need to obtain permission from FDA. Credit to the U.S. Food and Drug Administration as the source is appreciated but not required. | |
Retrosynthesis Analysis
AI-Powered Synthesis Planning: Our tool employs the Template_relevance Pistachio, Template_relevance Bkms_metabolic, Template_relevance Pistachio_ringbreaker, Template_relevance Reaxys, Template_relevance Reaxys_biocatalysis model, leveraging a vast database of chemical reactions to predict feasible synthetic routes.
One-Step Synthesis Focus: Specifically designed for one-step synthesis, it provides concise and direct routes for your target compounds, streamlining the synthesis process.
Accurate Predictions: Utilizing the extensive PISTACHIO, BKMS_METABOLIC, PISTACHIO_RINGBREAKER, REAXYS, REAXYS_BIOCATALYSIS database, our tool offers high-accuracy predictions, reflecting the latest in chemical research and data.
Strategy Settings
Precursor scoring | Relevance Heuristic |
---|---|
Min. plausibility | 0.01 |
Model | Template_relevance |
Template Set | Pistachio/Bkms_metabolic/Pistachio_ringbreaker/Reaxys/Reaxys_biocatalysis |
Top-N result to add to graph | 6 |
Feasible Synthetic Routes
Disclaimer and Information on In-Vitro Research Products
Please be aware that all articles and product information presented on BenchChem are intended solely for informational purposes. The products available for purchase on BenchChem are specifically designed for in-vitro studies, which are conducted outside of living organisms. In-vitro studies, derived from the Latin term "in glass," involve experiments performed in controlled laboratory settings using cells or tissues. It is important to note that these products are not categorized as medicines or drugs, and they have not received approval from the FDA for the prevention, treatment, or cure of any medical condition, ailment, or disease. We must emphasize that any form of bodily introduction of these products into humans or animals is strictly prohibited by law. It is essential to adhere to these guidelines to ensure compliance with legal and ethical standards in research and experimentation.