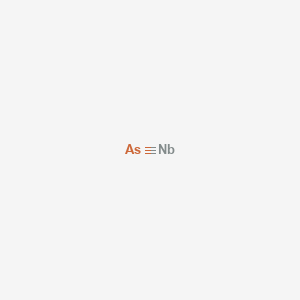
Niobium arsenide
Overview
Description
Niobium arsenide is a crystalline solid compound composed of niobium and arsenic. It is known for its unique properties as a semiconductor and its applications in photo-optic technologies. The compound has a body-centered tetragonal Bravais lattice structure and is classified as a Weyl semimetal, which exhibits distinct quantum and topological attributes .
Preparation Methods
Synthetic Routes and Reaction Conditions: Niobium arsenide can be synthesized through various methods, including solid-state reactions and chemical vapor deposition. One common method involves the direct reaction of niobium and arsenic at high temperatures in a controlled atmosphere to prevent oxidation. The reaction can be represented as:
Nb+As→NbAs
Industrial Production Methods: In industrial settings, this compound is often produced using high-purity niobium and arsenic sources. The materials are heated in a vacuum or inert gas environment to achieve the desired reaction. The resulting product is then purified through processes such as zone refining to obtain high-purity this compound suitable for semiconductor applications .
Chemical Reactions Analysis
Types of Reactions: Niobium arsenide undergoes various chemical reactions, including oxidation, reduction, and substitution. For example, it can react with halogens to form niobium halides and arsenic halides. The reaction with fluorine is particularly vigorous:
NbAs+F2→NbF5+AsF3
Common Reagents and Conditions:
Oxidation: this compound can be oxidized in the presence of oxygen at elevated temperatures to form niobium pentoxide and arsenic trioxide.
Reduction: It can be reduced using hydrogen gas to form elemental niobium and arsenic.
Substitution: this compound can undergo substitution reactions with other metal halides to form mixed-metal arsenides.
Major Products: The major products formed from these reactions include niobium pentoxide, arsenic trioxide, niobium halides, and arsenic halides .
Scientific Research Applications
Niobium arsenide has a wide range of scientific research applications due to its unique electronic properties. Some of its notable applications include:
Semiconductors: Used in the development of advanced semiconductor devices due to its high electron mobility and unique electronic structure.
Photo-optic Applications: Utilized in the production of lasers, light-emitting diodes, and optical sensors.
Quantum Computing: Its Weyl semimetal properties make it a candidate for quantum computing applications, where it can be used to study exotic quantum phenomena.
Pressure Sensors: Employed in the development of high-precision pressure sensors due to its sensitivity to external perturbations .
Mechanism of Action
The mechanism of action of niobium arsenide is primarily related to its electronic structure. As a Weyl semimetal, it features Weyl nodes in its band structure, which are associated with chiral charges. These nodes lead to unique electronic properties, such as high electron mobility and the presence of Fermi arcs on the surface. These properties enable this compound to exhibit unusual transport phenomena and make it suitable for applications in advanced electronic and quantum devices .
Comparison with Similar Compounds
Niobium arsenide can be compared with other similar compounds, such as:
Niobium phosphide: Another Weyl semimetal with similar electronic properties but different chemical reactivity.
Tantalum arsenide: Exhibits similar electronic structure and applications but has different physical properties due to the presence of tantalum.
Indium arsenide: A well-known semiconductor with high electron mobility, used in high-speed electronic devices.
Uniqueness: this compound’s uniqueness lies in its combination of high electron mobility, Weyl semimetal properties, and its ability to form stable compounds with various elements. This makes it a versatile material for a wide range of scientific and industrial applications .
Properties
IUPAC Name |
arsanylidyneniobium | |
---|---|---|
Source | PubChem | |
URL | https://pubchem.ncbi.nlm.nih.gov | |
Description | Data deposited in or computed by PubChem | |
InChI |
InChI=1S/As.Nb | |
Source | PubChem | |
URL | https://pubchem.ncbi.nlm.nih.gov | |
Description | Data deposited in or computed by PubChem | |
InChI Key |
RDSGSPSSNTWRJB-UHFFFAOYSA-N | |
Source | PubChem | |
URL | https://pubchem.ncbi.nlm.nih.gov | |
Description | Data deposited in or computed by PubChem | |
Canonical SMILES |
[As]#[Nb] | |
Source | PubChem | |
URL | https://pubchem.ncbi.nlm.nih.gov | |
Description | Data deposited in or computed by PubChem | |
Molecular Formula |
AsNb | |
Source | PubChem | |
URL | https://pubchem.ncbi.nlm.nih.gov | |
Description | Data deposited in or computed by PubChem | |
DSSTOX Substance ID |
DTXSID701314003 | |
Record name | Niobium arsenide (NbAs) | |
Source | EPA DSSTox | |
URL | https://comptox.epa.gov/dashboard/DTXSID701314003 | |
Description | DSSTox provides a high quality public chemistry resource for supporting improved predictive toxicology. | |
Molecular Weight |
167.82797 g/mol | |
Source | PubChem | |
URL | https://pubchem.ncbi.nlm.nih.gov | |
Description | Data deposited in or computed by PubChem | |
CAS No. |
12255-08-2 | |
Record name | Niobium arsenide (NbAs) | |
Source | CAS Common Chemistry | |
URL | https://commonchemistry.cas.org/detail?cas_rn=12255-08-2 | |
Description | CAS Common Chemistry is an open community resource for accessing chemical information. Nearly 500,000 chemical substances from CAS REGISTRY cover areas of community interest, including common and frequently regulated chemicals, and those relevant to high school and undergraduate chemistry classes. This chemical information, curated by our expert scientists, is provided in alignment with our mission as a division of the American Chemical Society. | |
Explanation | The data from CAS Common Chemistry is provided under a CC-BY-NC 4.0 license, unless otherwise stated. | |
Record name | Niobium arsenide (NbAs) | |
Source | ChemIDplus | |
URL | https://pubchem.ncbi.nlm.nih.gov/substance/?source=chemidplus&sourceid=0012255082 | |
Description | ChemIDplus is a free, web search system that provides access to the structure and nomenclature authority files used for the identification of chemical substances cited in National Library of Medicine (NLM) databases, including the TOXNET system. | |
Record name | Niobium arsenide (NbAs) | |
Source | EPA Chemicals under the TSCA | |
URL | https://www.epa.gov/chemicals-under-tsca | |
Description | EPA Chemicals under the Toxic Substances Control Act (TSCA) collection contains information on chemicals and their regulations under TSCA, including non-confidential content from the TSCA Chemical Substance Inventory and Chemical Data Reporting. | |
Record name | Niobium arsenide (NbAs) | |
Source | EPA DSSTox | |
URL | https://comptox.epa.gov/dashboard/DTXSID701314003 | |
Description | DSSTox provides a high quality public chemistry resource for supporting improved predictive toxicology. | |
Record name | Niobium arsenide | |
Source | European Chemicals Agency (ECHA) | |
URL | https://echa.europa.eu/substance-information/-/substanceinfo/100.032.263 | |
Description | The European Chemicals Agency (ECHA) is an agency of the European Union which is the driving force among regulatory authorities in implementing the EU's groundbreaking chemicals legislation for the benefit of human health and the environment as well as for innovation and competitiveness. | |
Explanation | Use of the information, documents and data from the ECHA website is subject to the terms and conditions of this Legal Notice, and subject to other binding limitations provided for under applicable law, the information, documents and data made available on the ECHA website may be reproduced, distributed and/or used, totally or in part, for non-commercial purposes provided that ECHA is acknowledged as the source: "Source: European Chemicals Agency, http://echa.europa.eu/". Such acknowledgement must be included in each copy of the material. ECHA permits and encourages organisations and individuals to create links to the ECHA website under the following cumulative conditions: Links can only be made to webpages that provide a link to the Legal Notice page. | |
Disclaimer and Information on In-Vitro Research Products
Please be aware that all articles and product information presented on BenchChem are intended solely for informational purposes. The products available for purchase on BenchChem are specifically designed for in-vitro studies, which are conducted outside of living organisms. In-vitro studies, derived from the Latin term "in glass," involve experiments performed in controlled laboratory settings using cells or tissues. It is important to note that these products are not categorized as medicines or drugs, and they have not received approval from the FDA for the prevention, treatment, or cure of any medical condition, ailment, or disease. We must emphasize that any form of bodily introduction of these products into humans or animals is strictly prohibited by law. It is essential to adhere to these guidelines to ensure compliance with legal and ethical standards in research and experimentation.