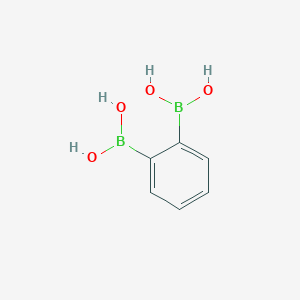
1,2-Phenylenediboronic acid
Overview
Description
1,2-Phenylenediboronic acid is an organic compound containing two boronic acid groups attached to adjacent carbon atoms on a benzene ring.
Mechanism of Action
Target of Action
1,2-Phenylenediboronic acid has been investigated as a potential catalyst for organic synthesis . It has also been studied as a potential inhibitor of β-lactamase enzymes, specifically KPC-type carbapenemases .
Mode of Action
In the context of organic synthesis, this compound acts as a bidentate Lewis acid, catalyzing the inverse electron demand Diels–Alder reaction of 1,2-diazene . As a β-lactamase inhibitor, it forms a complex with the KPC-2 enzyme, enabling covalent binding to the catalytic Ser70 residue .
Biochemical Pathways
Its role as a catalyst in the inverse electron demand diels–alder reaction suggests it may influence pathways involving 1,2-diazene . As a β-lactamase inhibitor, it likely affects pathways related to bacterial resistance to β-lactam antibiotics .
Pharmacokinetics
Its potential as a β-lactamase inhibitor suggests it may have suitable bioavailability for therapeutic use .
Result of Action
As a catalyst, this compound facilitates the inverse electron demand Diels–Alder reaction of 1,2-diazene . As a β-lactamase inhibitor, it reduces the minimum inhibitory concentrations (MICs) of carbapenems, enhancing their effectiveness against KPC-producers .
Action Environment
The action of this compound can be influenced by environmental factors. For instance, its stability and ease of handling make it a more practical catalyst than some alternatives . Its effectiveness as a β-lactamase inhibitor may also be influenced by factors such as bacterial resistance mechanisms .
Biochemical Analysis
Biochemical Properties
1,2-Phenylenediboronic acid has been investigated as a potential catalyst for biochemical transformations . It has been found to interact with various biomolecules, including enzymes and proteins, in a bidentate coordination mode . This interaction is crucial for its catalytic activity .
Cellular Effects
For instance, certain aromatic diboronic acids have been found to be effective inhibitors of KPC/AmpC, enzymes that are involved in antibiotic resistance .
Molecular Mechanism
The molecular mechanism of this compound involves its ability to form bidentate coordination complexes with biomolecules . This enables it to exert its effects at the molecular level, potentially influencing enzyme activity and gene expression .
Temporal Effects in Laboratory Settings
The stability of this compound makes it suitable for long-term studies in laboratory settings . Specific information on its degradation and long-term effects on cellular function is currently limited.
Preparation Methods
Synthetic Routes and Reaction Conditions
1,2-Phenylenediboronic acid can be synthesized through several methods. One common approach involves the metal exchange reaction of 1,2-bis(trimethylsilyl)benzene with tetrachloro-1,2-bisborobenzene, followed by the exchange of chlorides with 1,2-diols . This method typically involves the use of an iron-catalyzed Grignard reaction to produce the intermediate compounds, which are then converted to the final boronic ester .
Industrial Production Methods
Industrial production methods for this compound are not extensively documented. the synthesis methods used in research laboratories can be scaled up for industrial applications, provided that the reaction conditions are optimized for larger-scale production .
Chemical Reactions Analysis
Types of Reactions
1,2-Phenylenediboronic acid undergoes various chemical reactions, including:
Oxidation: This compound can be oxidized to form corresponding boronic esters or acids.
Reduction: Reduction reactions can convert the boronic acid groups to other functional groups.
Substitution: The boronic acid groups can participate in substitution reactions, such as the Suzuki-Miyaura cross-coupling reaction.
Common Reagents and Conditions
Common reagents used in reactions involving this compound include palladium catalysts for cross-coupling reactions, oxidizing agents for oxidation reactions, and reducing agents for reduction reactions .
Major Products
The major products formed from these reactions depend on the specific reaction conditions and reagents used. For example, the Suzuki-Miyaura cross-coupling reaction typically produces biaryl compounds .
Scientific Research Applications
1,2-Phenylenediboronic acid has several scientific research applications:
Comparison with Similar Compounds
Similar Compounds
1,4-Phenylenediboronic acid: Similar in structure but with boronic acid groups at the para positions.
Benzene-1,2,4-triboronic acid: Contains three boronic acid groups and is used in more complex catalytic systems.
Boronated thiophenes: These compounds have boronic acid groups attached to thiophene rings and are used in optoelectronic devices.
Uniqueness
1,2-Phenylenediboronic acid is unique due to its ortho configuration, which allows for the formation of stable bidentate coordination complexes. This property makes it particularly effective as a catalyst in certain reactions, offering higher stability and easier handling compared to other boronic acid derivatives .
Biological Activity
1,2-Phenylenediboronic acid is a member of the phenylboronic acid family that has garnered attention for its potential biological activities, particularly in the context of antibiotic resistance and cancer treatment. This compound exhibits significant interactions with various biological targets, making it a candidate for further research in medicinal chemistry and pharmacology.
Inhibition of β-Lactamases
Recent studies have highlighted the effectiveness of this compound as an inhibitor of KPC-type carbapenemases, which are critical in bacterial resistance to last-resort antibiotics. The compound demonstrated the highest activity among various isomers, significantly reducing the minimum inhibitory concentrations (MICs) of carbapenems by up to 16-fold at a concentration of 8/4 mg/L. Checkerboard assays indicated strong synergy between this compound and carbapenems, with fractional inhibitory concentration indices ranging from 0.1 to 0.32, suggesting a potent combined effect against resistant strains .
The mechanism underlying the inhibition involves the covalent binding of this compound to the active site of KPC enzymes. Quantum mechanics/molecular mechanics calculations have modeled this interaction, revealing that the compound effectively binds to the catalytic Ser70 residue of KPC-2, thereby obstructing its enzymatic activity .
Antifungal Activity
In addition to its antibacterial properties, this compound has shown moderate antifungal activity against several Candida species. The minimum fungicidal concentrations (MFCs) ranged from 400 mg/L for certain derivatives against Candida albicans and related species . This dual action against both bacterial and fungal pathogens positions it as a versatile agent in antimicrobial therapy.
Structural Properties and Acidity
The structural characteristics of this compound contribute to its biological activity. The compound exhibits high acidity due to the proximity of two boronic groups, which enhances its electrophilic character. This property is crucial for its interaction with biological targets and influences its reactivity in various chemical environments .
Data Table: Biological Activity Summary
Biological Activity | Target Organisms | MIC/MFC Values | Notes |
---|---|---|---|
KPC-type Carbapenemases | E. coli (KPC-3) | MIC reduction up to 16-fold | Synergistic effect with carbapenems |
AmpC-type β-lactamases | Various bacteria | MICs comparable to controls | Enhanced ceftazidime activity |
Fungal Infections | Candida albicans | MFC = 400 mg/L | Moderate antifungal activity |
Case Study 1: Efficacy Against KPC-Producing Strains
A recent clinical study evaluated the efficacy of this compound in combination with carbapenems against KPC-producing E. coli. The results demonstrated a significant reduction in bacterial load in vitro and improved outcomes in animal models when treated with this combination therapy compared to carbapenems alone .
Case Study 2: Antifungal Properties
Another investigation focused on the antifungal properties of this compound derivatives against Candida species. The study found that certain modifications to the boronic acid structure enhanced antifungal efficacy, suggesting that further chemical optimization could yield more potent antifungal agents .
Properties
IUPAC Name |
(2-boronophenyl)boronic acid | |
---|---|---|
Source | PubChem | |
URL | https://pubchem.ncbi.nlm.nih.gov | |
Description | Data deposited in or computed by PubChem | |
InChI |
InChI=1S/C6H8B2O4/c9-7(10)5-3-1-2-4-6(5)8(11)12/h1-4,9-12H | |
Source | PubChem | |
URL | https://pubchem.ncbi.nlm.nih.gov | |
Description | Data deposited in or computed by PubChem | |
InChI Key |
ZKRVXVDQQOVOIM-UHFFFAOYSA-N | |
Source | PubChem | |
URL | https://pubchem.ncbi.nlm.nih.gov | |
Description | Data deposited in or computed by PubChem | |
Canonical SMILES |
B(C1=CC=CC=C1B(O)O)(O)O | |
Source | PubChem | |
URL | https://pubchem.ncbi.nlm.nih.gov | |
Description | Data deposited in or computed by PubChem | |
Molecular Formula |
C6H8B2O4 | |
Source | PubChem | |
URL | https://pubchem.ncbi.nlm.nih.gov | |
Description | Data deposited in or computed by PubChem | |
DSSTOX Substance ID |
DTXSID00623233 | |
Record name | 1,2-Phenylenediboronic acid | |
Source | EPA DSSTox | |
URL | https://comptox.epa.gov/dashboard/DTXSID00623233 | |
Description | DSSTox provides a high quality public chemistry resource for supporting improved predictive toxicology. | |
Molecular Weight |
165.75 g/mol | |
Source | PubChem | |
URL | https://pubchem.ncbi.nlm.nih.gov | |
Description | Data deposited in or computed by PubChem | |
CAS No. |
13506-83-7 | |
Record name | B,B′-1,2-Phenylenebis[boronic acid] | |
Source | CAS Common Chemistry | |
URL | https://commonchemistry.cas.org/detail?cas_rn=13506-83-7 | |
Description | CAS Common Chemistry is an open community resource for accessing chemical information. Nearly 500,000 chemical substances from CAS REGISTRY cover areas of community interest, including common and frequently regulated chemicals, and those relevant to high school and undergraduate chemistry classes. This chemical information, curated by our expert scientists, is provided in alignment with our mission as a division of the American Chemical Society. | |
Explanation | The data from CAS Common Chemistry is provided under a CC-BY-NC 4.0 license, unless otherwise stated. | |
Record name | 1,2-Phenylenediboronic acid | |
Source | EPA DSSTox | |
URL | https://comptox.epa.gov/dashboard/DTXSID00623233 | |
Description | DSSTox provides a high quality public chemistry resource for supporting improved predictive toxicology. | |
Q1: How does the unique structure of 1,2-phenylenediboronic acid influence its interactions and applications?
A1: this compound exhibits a propensity to form various supramolecular structures due to the presence of two boronic acid groups on the aromatic ring. [, , ] This feature facilitates the formation of cyclic semianhydrides, particularly in the presence of water or fluoride ions. [] Computational studies have shown that fluorination of the aromatic ring further stabilizes these cyclic structures. [] Furthermore, the molecule readily forms hydrogen-bonded networks, as exemplified by its ability to create nanotubular architectures capable of hosting water clusters. [] This self-assembling property makes it a promising candidate for applications in supramolecular chemistry and materials science, such as the design of hydrogen-bonded organic frameworks (HOFs). []
Q2: Can this compound be utilized in the development of luminescent materials?
A2: Yes, this compound can be complexed with appropriate ligands to create luminescent materials. Studies have shown its ability to form complexes with imidazo[1,2-a]pyridine derivatives, yielding blue-luminescent compounds. [] These complexes exhibit characteristic dimeric structures linked by the boronic acid groups, influencing their luminescent properties. [] The lack of significant charge transfer within these complexes, unlike some analogous systems, suggests that their emission properties are primarily governed by the chosen ligand. [] This finding highlights the potential of this compound as a building block for designing tailored luminescent materials by selecting suitable ligands.
Q3: How do substituents on the aromatic ring of this compound affect its reactivity?
A3: The reactivity of this compound can be significantly influenced by substituents on the aromatic ring. For instance, incorporating bromine atoms at the 2 and 6 positions facilitates a two-step synthesis of DATB (1,3-dioxa-5-aza-2,4,6-triborinane) derivatives. [] In this process, the brominated this compound undergoes Suzuki-Miyaura coupling followed by an acidic treatment to yield the desired DATB ring system. [] This example demonstrates how modifications to the parent this compound structure can be strategically employed to access other valuable heterocyclic compounds.
Disclaimer and Information on In-Vitro Research Products
Please be aware that all articles and product information presented on BenchChem are intended solely for informational purposes. The products available for purchase on BenchChem are specifically designed for in-vitro studies, which are conducted outside of living organisms. In-vitro studies, derived from the Latin term "in glass," involve experiments performed in controlled laboratory settings using cells or tissues. It is important to note that these products are not categorized as medicines or drugs, and they have not received approval from the FDA for the prevention, treatment, or cure of any medical condition, ailment, or disease. We must emphasize that any form of bodily introduction of these products into humans or animals is strictly prohibited by law. It is essential to adhere to these guidelines to ensure compliance with legal and ethical standards in research and experimentation.