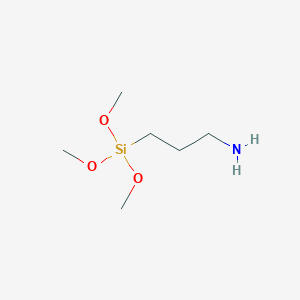
3-Aminopropyltrimethoxysilane
Overview
Description
3-Aminopropyltrimethoxysilane: is an organosilicon compound with the chemical formula C6H17NO3Si . It is a colorless liquid that is widely used as a silane coupling agent and surface modifier. This compound is particularly valued for its ability to introduce amino groups onto surfaces, which can then be used for further chemical modifications.
Mechanism of Action
Target of Action
3-Aminopropyltrimethoxysilane (APTMS) is primarily targeted towards metal oxide nanoparticle (MONP) surfaces . It is used as a silane coupling agent for the surface modification of a variety of nanomaterials .
Mode of Action
APTMS functionalizes substrates with alkoxysilane molecules . It is frequently used in the process of silanization, which involves the functionalization of surfaces with alkoxysilane molecules . It can also be used for covalently attaching organic films to metal oxides such as silica and titania .
Biochemical Pathways
The biochemical pathways affected by APTMS are primarily related to the surface modification of nanomaterials . The introduction of amine (NH2) enhances their dispersibility and anti-bacterial property .
Pharmacokinetics
It’s known that aptms forms a thermally stable layer on different substrates .
Result of Action
The result of APTMS action is the creation of a thermally stable layer on different substrates . This layer enhances the dispersibility and anti-bacterial property of the substrates . It also allows for the covalent attachment of organic films to metal oxides .
Biochemical Analysis
Biochemical Properties
3-Aminopropyltrimethoxysilane plays a significant role in biochemical reactions, particularly in the process of silanization, the functionalization of surfaces with alkoxysilane molecules . It can also be used for covalent attaching of organic films to metal oxides such as silica and titania
Cellular Effects
The cellular effects of this compound are primarily observed in its role as a silane coupling agent for surface modification of nanomaterials . It has been shown to form a thermally stable layer on different substrates
Molecular Mechanism
The molecular mechanism of this compound involves its role as a catalyst in sol–gel silica polymerization . It is also employed as the functional monomer to create imprinted nanocavities for specific recognition of target molecules . The as-synthesized molecularly imprinted polymers (MIPs) exhibit ultra-high recognition capability .
Temporal Effects in Laboratory Settings
It is known that this compound-based films form a thermally stable layer on different substrates , suggesting potential long-term stability.
Preparation Methods
Synthetic Routes and Reaction Conditions: 3-Aminopropyltrimethoxysilane can be synthesized through the reaction of 3-chloropropyltrimethoxysilane with ammonia or an amine . The reaction typically occurs under mild conditions, often at room temperature, and involves the substitution of the chlorine atom with an amino group.
Industrial Production Methods: In industrial settings, this compound is produced by reacting 3-chloropropyltrimethoxysilane with excess ammonia in a solvent such as methanol . The reaction is carried out in a sealed reactor to prevent the escape of ammonia gas. The product is then purified through distillation to remove any unreacted starting materials and by-products.
Chemical Reactions Analysis
Types of Reactions:
Substitution Reactions: 3-Aminopropyltrimethoxysilane can undergo substitution reactions where the methoxy groups are replaced by other functional groups.
Condensation Reactions: It can participate in condensation reactions with other silanes or silanols, forming siloxane bonds.
Hydrolysis: In the presence of water, this compound hydrolyzes to form silanols, which can further condense to form siloxane networks.
Common Reagents and Conditions:
Water: Used for hydrolysis reactions.
Alcohols: Often used as solvents in various reactions.
Acids or Bases: Can be used to catalyze hydrolysis and condensation reactions.
Major Products:
Siloxane Networks: Formed through condensation reactions.
Functionalized Surfaces:
Scientific Research Applications
Chemistry: 3-Aminopropyltrimethoxysilane is used as a coupling agent to improve the adhesion between organic and inorganic materials. It is also used in the synthesis of hybrid materials and nanocomposites.
Biology: In biological research, this compound is used to functionalize surfaces for the immobilization of biomolecules, such as proteins and DNA, which is essential for biosensor development.
Medicine: It is used in the development of drug delivery systems and medical implants. The amino groups on the surface can be used to attach therapeutic agents or to modify the surface properties of implants to improve biocompatibility.
Industry: this compound is used in the production of coatings, adhesives, and sealants. It is also used in the modification of glass and metal surfaces to improve their chemical resistance and mechanical properties.
Comparison with Similar Compounds
- 3-Aminopropyltriethoxysilane
- N-(2-aminoethyl)-3-aminopropyltrimethoxysilane
- 3-Mercaptopropyltrimethoxysilane
Comparison: 3-Aminopropyltrimethoxysilane is unique in its balance of reactivity and stability. Compared to 3-Aminopropyltriethoxysilane, it has a higher reactivity due to the presence of methoxy groups, which hydrolyze more readily than ethoxy groups. This makes it more suitable for applications requiring rapid surface modification. N-(2-aminoethyl)-3-aminopropyltrimethoxysilane has an additional amino group, providing more sites for further functionalization, but it may also introduce more complexity in the reaction process. 3-Mercaptopropyltrimethoxysilane, on the other hand, introduces thiol groups instead of amino groups, which can be useful for different types of surface modifications and applications.
Properties
IUPAC Name |
3-trimethoxysilylpropan-1-amine | |
---|---|---|
Source | PubChem | |
URL | https://pubchem.ncbi.nlm.nih.gov | |
Description | Data deposited in or computed by PubChem | |
InChI |
InChI=1S/C6H17NO3Si/c1-8-11(9-2,10-3)6-4-5-7/h4-7H2,1-3H3 | |
Source | PubChem | |
URL | https://pubchem.ncbi.nlm.nih.gov | |
Description | Data deposited in or computed by PubChem | |
InChI Key |
SJECZPVISLOESU-UHFFFAOYSA-N | |
Source | PubChem | |
URL | https://pubchem.ncbi.nlm.nih.gov | |
Description | Data deposited in or computed by PubChem | |
Canonical SMILES |
CO[Si](CCCN)(OC)OC | |
Source | PubChem | |
URL | https://pubchem.ncbi.nlm.nih.gov | |
Description | Data deposited in or computed by PubChem | |
Molecular Formula |
C6H17NO3Si | |
Source | PubChem | |
URL | https://pubchem.ncbi.nlm.nih.gov | |
Description | Data deposited in or computed by PubChem | |
Related CAS |
54115-51-4 | |
Record name | 3-Aminopropyltrimethoxysilane homopolymer | |
Source | CAS Common Chemistry | |
URL | https://commonchemistry.cas.org/detail?cas_rn=54115-51-4 | |
Description | CAS Common Chemistry is an open community resource for accessing chemical information. Nearly 500,000 chemical substances from CAS REGISTRY cover areas of community interest, including common and frequently regulated chemicals, and those relevant to high school and undergraduate chemistry classes. This chemical information, curated by our expert scientists, is provided in alignment with our mission as a division of the American Chemical Society. | |
Explanation | The data from CAS Common Chemistry is provided under a CC-BY-NC 4.0 license, unless otherwise stated. | |
DSSTOX Substance ID |
DTXSID0038833 | |
Record name | 3-(Trimethoxysilyl)propan-1-amine | |
Source | EPA DSSTox | |
URL | https://comptox.epa.gov/dashboard/DTXSID0038833 | |
Description | DSSTox provides a high quality public chemistry resource for supporting improved predictive toxicology. | |
Molecular Weight |
179.29 g/mol | |
Source | PubChem | |
URL | https://pubchem.ncbi.nlm.nih.gov | |
Description | Data deposited in or computed by PubChem | |
Physical Description |
Liquid, Clear to straw-colored liquid with an ammoniacal odor; [Gelest MSDS] | |
Record name | 1-Propanamine, 3-(trimethoxysilyl)- | |
Source | EPA Chemicals under the TSCA | |
URL | https://www.epa.gov/chemicals-under-tsca | |
Description | EPA Chemicals under the Toxic Substances Control Act (TSCA) collection contains information on chemicals and their regulations under TSCA, including non-confidential content from the TSCA Chemical Substance Inventory and Chemical Data Reporting. | |
Record name | 3-Aminopropyltrimethoxysilane | |
Source | Haz-Map, Information on Hazardous Chemicals and Occupational Diseases | |
URL | https://haz-map.com/Agents/11018 | |
Description | Haz-Map® is an occupational health database designed for health and safety professionals and for consumers seeking information about the adverse effects of workplace exposures to chemical and biological agents. | |
Explanation | Copyright (c) 2022 Haz-Map(R). All rights reserved. Unless otherwise indicated, all materials from Haz-Map are copyrighted by Haz-Map(R). No part of these materials, either text or image may be used for any purpose other than for personal use. Therefore, reproduction, modification, storage in a retrieval system or retransmission, in any form or by any means, electronic, mechanical or otherwise, for reasons other than personal use, is strictly prohibited without prior written permission. | |
CAS No. |
13822-56-5 | |
Record name | Aminopropyltrimethoxysilane | |
Source | CAS Common Chemistry | |
URL | https://commonchemistry.cas.org/detail?cas_rn=13822-56-5 | |
Description | CAS Common Chemistry is an open community resource for accessing chemical information. Nearly 500,000 chemical substances from CAS REGISTRY cover areas of community interest, including common and frequently regulated chemicals, and those relevant to high school and undergraduate chemistry classes. This chemical information, curated by our expert scientists, is provided in alignment with our mission as a division of the American Chemical Society. | |
Explanation | The data from CAS Common Chemistry is provided under a CC-BY-NC 4.0 license, unless otherwise stated. | |
Record name | 3-Aminopropyltrimethoxysilane | |
Source | ChemIDplus | |
URL | https://pubchem.ncbi.nlm.nih.gov/substance/?source=chemidplus&sourceid=0013822565 | |
Description | ChemIDplus is a free, web search system that provides access to the structure and nomenclature authority files used for the identification of chemical substances cited in National Library of Medicine (NLM) databases, including the TOXNET system. | |
Record name | 3-Aminopropyltrimethoxysilane | |
Source | DTP/NCI | |
URL | https://dtp.cancer.gov/dtpstandard/servlet/dwindex?searchtype=NSC&outputformat=html&searchlist=83845 | |
Description | The NCI Development Therapeutics Program (DTP) provides services and resources to the academic and private-sector research communities worldwide to facilitate the discovery and development of new cancer therapeutic agents. | |
Explanation | Unless otherwise indicated, all text within NCI products is free of copyright and may be reused without our permission. Credit the National Cancer Institute as the source. | |
Record name | 1-Propanamine, 3-(trimethoxysilyl)- | |
Source | EPA Chemicals under the TSCA | |
URL | https://www.epa.gov/chemicals-under-tsca | |
Description | EPA Chemicals under the Toxic Substances Control Act (TSCA) collection contains information on chemicals and their regulations under TSCA, including non-confidential content from the TSCA Chemical Substance Inventory and Chemical Data Reporting. | |
Record name | 3-(Trimethoxysilyl)propan-1-amine | |
Source | EPA DSSTox | |
URL | https://comptox.epa.gov/dashboard/DTXSID0038833 | |
Description | DSSTox provides a high quality public chemistry resource for supporting improved predictive toxicology. | |
Record name | 3-(trimethoxysilyl)propylamine | |
Source | European Chemicals Agency (ECHA) | |
URL | https://echa.europa.eu/substance-information/-/substanceinfo/100.034.087 | |
Description | The European Chemicals Agency (ECHA) is an agency of the European Union which is the driving force among regulatory authorities in implementing the EU's groundbreaking chemicals legislation for the benefit of human health and the environment as well as for innovation and competitiveness. | |
Explanation | Use of the information, documents and data from the ECHA website is subject to the terms and conditions of this Legal Notice, and subject to other binding limitations provided for under applicable law, the information, documents and data made available on the ECHA website may be reproduced, distributed and/or used, totally or in part, for non-commercial purposes provided that ECHA is acknowledged as the source: "Source: European Chemicals Agency, http://echa.europa.eu/". Such acknowledgement must be included in each copy of the material. ECHA permits and encourages organisations and individuals to create links to the ECHA website under the following cumulative conditions: Links can only be made to webpages that provide a link to the Legal Notice page. | |
Record name | 3-(TRIMETHOXYSILYL)PROPYLAMINE | |
Source | FDA Global Substance Registration System (GSRS) | |
URL | https://gsrs.ncats.nih.gov/ginas/app/beta/substances/7MRY0C9SB6 | |
Description | The FDA Global Substance Registration System (GSRS) enables the efficient and accurate exchange of information on what substances are in regulated products. Instead of relying on names, which vary across regulatory domains, countries, and regions, the GSRS knowledge base makes it possible for substances to be defined by standardized, scientific descriptions. | |
Explanation | Unless otherwise noted, the contents of the FDA website (www.fda.gov), both text and graphics, are not copyrighted. They are in the public domain and may be republished, reprinted and otherwise used freely by anyone without the need to obtain permission from FDA. Credit to the U.S. Food and Drug Administration as the source is appreciated but not required. | |
Synthesis routes and methods I
Procedure details
Synthesis routes and methods II
Procedure details
Retrosynthesis Analysis
AI-Powered Synthesis Planning: Our tool employs the Template_relevance Pistachio, Template_relevance Bkms_metabolic, Template_relevance Pistachio_ringbreaker, Template_relevance Reaxys, Template_relevance Reaxys_biocatalysis model, leveraging a vast database of chemical reactions to predict feasible synthetic routes.
One-Step Synthesis Focus: Specifically designed for one-step synthesis, it provides concise and direct routes for your target compounds, streamlining the synthesis process.
Accurate Predictions: Utilizing the extensive PISTACHIO, BKMS_METABOLIC, PISTACHIO_RINGBREAKER, REAXYS, REAXYS_BIOCATALYSIS database, our tool offers high-accuracy predictions, reflecting the latest in chemical research and data.
Strategy Settings
Precursor scoring | Relevance Heuristic |
---|---|
Min. plausibility | 0.01 |
Model | Template_relevance |
Template Set | Pistachio/Bkms_metabolic/Pistachio_ringbreaker/Reaxys/Reaxys_biocatalysis |
Top-N result to add to graph | 6 |
Feasible Synthetic Routes
A: APTMS primarily interacts with surfaces possessing hydroxyl groups, like silica [, , ] or alumina []. This interaction occurs through a process called silanization, where the hydrolyzed silanol groups of APTMS condense with surface hydroxyl groups, forming stable Si-O-Si bonds [, , ]. This effectively anchors the aminopropyl group of APTMS to the surface, introducing amine functionality [, , , ]. This surface modification can lead to various downstream effects, such as altered hydrophilicity [, ], improved adhesion [, ], and the ability to bind to other molecules like dyes [] or enzymes [].
ANone:
- Spectroscopic Data:
- FTIR: Characteristic peaks are observed for N-H stretching (3300–3500 cm−1), C-H stretching (2800–3000 cm−1), Si-O-Si stretching (1000–1100 cm−1), and Si-C stretching (750–850 cm−1) [, , , ].
- NMR:
- XPS: Used to confirm the presence of silicon, nitrogen, and oxygen, and analyze the chemical environment of these elements on modified surfaces [, ].
A: While APTMS itself is not a catalyst, it plays a crucial role in heterogeneous catalysis by acting as a linker for immobilizing catalytic species onto solid supports []. For instance, it facilitates the anchoring of enzymes like chloroperoxidase [] and glucose oxidase [] onto mesoporous silica materials like SBA-15, enabling their use in continuous flow reactors. Additionally, APTMS can modify the surface properties of catalysts, influencing their activity and selectivity [].
A: Molecular dynamics simulations have been employed to investigate the interaction of APTMS with polymers like epoxy resin []. These simulations help understand the effect of APTMS on the thermo-mechanical properties of the composite material at a molecular level []. DFT calculations aid in interpreting spectroscopic data, like inelastic neutron scattering spectra, to gain insights into the intermolecular interactions of APTMS [].
A: Modifying the structure of APTMS can significantly affect its properties and applications. For instance, increasing the alkyl chain length between the amine and silane groups can improve the oil resistance of rubber composites [, ]. Using different aminosilanes with varying amine groups (mono-, di-, or triamine) can impact the separation efficiency in chromatographic applications [].
A: APTMS is typically used as a solution in organic solvents like toluene or ethanol []. The stability of these solutions is influenced by factors like moisture and storage conditions. Strategies to improve hydrolytic stability involve optimizing deposition conditions, such as using anhydrous solvents and controlling the reaction time [].
A: Several alternatives to APTMS exist, including other aminosilanes like 3-aminopropyltriethoxysilane (APTES) [, ], N-(2-aminoethyl)-3-aminopropyltrimethoxysilane (AEAPTMS) [, , ], and 3-[2-(2-aminoethylamino)ethylamino]propyltrimethoxysilane [, ]. The choice of silane depends on the specific application and desired properties. For instance, APTES is often used interchangeably with APTMS, but the choice may depend on factors like hydrolysis rate and stability of the resulting layer []. Other surface modification techniques, like plasma treatment, can also be considered as alternatives.
ANone: While APTMS itself is not considered highly toxic, the production and disposal of APTMS-containing materials need careful consideration. Strategies for resource efficiency include optimizing reaction conditions to minimize waste and exploring the use of renewable resources in the synthesis of APTMS or its alternatives.
A: Research on APTMS benefits from access to a range of analytical techniques, including spectroscopy (FTIR, NMR, XPS) [, , , ], microscopy (SEM, TEM) [, ], and surface characterization tools [, ]. Computational resources for molecular modeling and simulations further enhance the understanding of APTMS behavior [].
A: APTMS research exemplifies cross-disciplinary collaboration, drawing expertise from chemistry, materials science, engineering, and biology. For example, its use in enzyme immobilization for biocatalysis [] requires knowledge from both chemistry and biotechnology. Similarly, its application in developing sensors for environmental monitoring [] involves contributions from chemistry, materials science, and environmental science.
Disclaimer and Information on In-Vitro Research Products
Please be aware that all articles and product information presented on BenchChem are intended solely for informational purposes. The products available for purchase on BenchChem are specifically designed for in-vitro studies, which are conducted outside of living organisms. In-vitro studies, derived from the Latin term "in glass," involve experiments performed in controlled laboratory settings using cells or tissues. It is important to note that these products are not categorized as medicines or drugs, and they have not received approval from the FDA for the prevention, treatment, or cure of any medical condition, ailment, or disease. We must emphasize that any form of bodily introduction of these products into humans or animals is strictly prohibited by law. It is essential to adhere to these guidelines to ensure compliance with legal and ethical standards in research and experimentation.