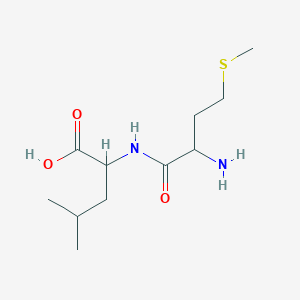
(S)-2-((S)-2-Amino-4-(methylthio)butanamido)-4-methylpentanoic acid
Overview
Description
Methionine-Leucine, commonly referred to as (S)-2-((S)-2-Amino-4-(methylthio)butanamido)-4-methylpentanoic acid, is a dipeptide composed of the amino acids methionine and leucine Methionine is an essential amino acid that plays a critical role in various metabolic processes, while leucine is a branched-chain amino acid important for protein synthesis and muscle repair
Mechanism of Action
Target of Action
The primary targets of Met-Leu are the PDE5A and PRKAB1 . PDE5A, or phosphodiesterase-5A, is an enzyme that plays a crucial role in regulating intracellular concentrations of cyclic guanosine monophosphate (cGMP). PRKAB1, or AMP-activated protein kinase subunit beta-1, is a component of the AMP-activated protein kinase (AMPK) system, which plays a key role in regulating cellular energy homeostasis .
Mode of Action
Met-Leu acts as a PDE5A inhibitor and a PRKAB1 activator . By inhibiting PDE5A, Met-Leu prevents the breakdown of cGMP, leading to increased cGMP levels. This can result in various physiological effects, including vasodilation and increased blood flow. As a PRKAB1 activator, Met-Leu stimulates the AMPK system, promoting energy production and utilization .
Biochemical Pathways
Met-Leu affects several biochemical pathways. It has been found to activate the AMPK/Sirt1 pathway , which plays a crucial role in energy metabolism and insulin sensitivity . Additionally, Met-Leu is involved in the biosynthesis of Leu and Met and the Met chain elongation cycle for glucosinolate formation .
Pharmacokinetics
Studies have shown that met-leu has arapid absorption and elimination rate
Result of Action
Met-Leu has been found to have several molecular and cellular effects. It can increase insulin sensitivity in skeletal muscle and stimulate glucose and lipid metabolism . Additionally, it has been shown to prevent cellular senescence and proteostasis disruption induced by myotube atrophy .
Action Environment
The action of Met-Leu can be influenced by various environmental factors. For instance, the presence of other compounds, such as sildenafil, can enhance the effects of Met-Leu . Additionally, the physiological state of the organism, such as age and metabolic status, can also affect the action, efficacy, and stability of Met-Leu .
Biochemical Analysis
Biochemical Properties
Methionine-Leucine interacts with various enzymes, proteins, and other biomolecules. For instance, methionine transporters have been harnessed for the production of methionine and other valuable biomolecules . These transporters are discussed with respect to their function, gene regulation, and biotechnological applications .
Cellular Effects
Methionine-Leucine influences cell function in several ways. It impacts cell signaling pathways, gene expression, and cellular metabolism. For example, methionine has been shown to be involved in the production of S-adenosyl-L-methionine, a universal methyl donor involved in the methylation of a variety of substrates, including DNA, RNA, proteins, and lipids .
Molecular Mechanism
At the molecular level, Methionine-Leucine exerts its effects through binding interactions with biomolecules, enzyme inhibition or activation, and changes in gene expression. For instance, methionine transporters of four families increase production yield and specificity .
Metabolic Pathways
Methionine-Leucine is involved in several metabolic pathways. It interacts with various enzymes or cofactors, and can also affect metabolic flux or metabolite levels. For example, methionine is involved in the methionine cycle, which is crucial for the synthesis of S-adenosylmethionine, a universal methyl donor .
Preparation Methods
Synthetic Routes and Reaction Conditions
The synthesis of Methionine-Leucine can be achieved through standard peptide synthesis techniques. One common method involves the use of solid-phase peptide synthesis (SPPS), where the amino acids are sequentially added to a growing peptide chain anchored to a solid resin. The process typically involves the following steps:
Activation: Methionine and leucine are activated using coupling reagents such as dicyclohexylcarbodiimide (DCC) or N,N’-diisopropylcarbodiimide (DIC) in the presence of a catalyst like 1-hydroxybenzotriazole (HOBt).
Coupling: The activated methionine is coupled to the resin-bound leucine under controlled conditions, usually in an inert atmosphere to prevent oxidation.
Deprotection: Protective groups on the amino acids are removed using reagents like trifluoroacetic acid (TFA) to yield the final dipeptide.
Industrial Production Methods
Industrial production of Methionine-Leucine can be scaled up using automated peptide synthesizers, which allow for high-throughput synthesis of peptides. The process involves similar steps as described above but is optimized for large-scale production. Additionally, microbial fermentation techniques can be employed, where genetically engineered microorganisms are used to produce the dipeptide in large quantities.
Chemical Reactions Analysis
Types of Reactions
Methionine-Leucine can undergo various chemical reactions, including:
Oxidation: Methionine residues can be oxidized to methionine sulfoxide or methionine sulfone using oxidizing agents like hydrogen peroxide (H₂O₂) or performic acid.
Reduction: Reduction of oxidized methionine residues can be achieved using reducing agents such as dithiothreitol (DTT) or tris(2-carboxyethyl)phosphine (TCEP).
Substitution: Methionine can participate in substitution reactions where the sulfur atom is replaced by other functional groups using reagents like iodoacetamide.
Common Reagents and Conditions
Oxidation: Hydrogen peroxide (H₂O₂), performic acid.
Reduction: Dithiothreitol (DTT), tris(2-carboxyethyl)phosphine (TCEP).
Substitution: Iodoacetamide.
Major Products Formed
Oxidation: Methionine sulfoxide, methionine sulfone.
Reduction: Methionine.
Substitution: Methionine derivatives with substituted functional groups.
Scientific Research Applications
Methionine-Leucine has a wide range of applications in scientific research, including:
Chemistry: Used as a model compound in studies of peptide synthesis and peptide bond formation.
Biology: Investigated for its role in protein-protein interactions and as a substrate for proteolytic enzymes.
Medicine: Explored for its potential therapeutic effects in muscle repair and as a nutritional supplement.
Industry: Utilized in the production of specialized peptides for research and pharmaceutical applications.
Comparison with Similar Compounds
Methionine-Leucine can be compared with other dipeptides and amino acid combinations, such as:
Methionine-Valine: Similar in structure but with valine instead of leucine, affecting its biochemical properties and applications.
Leucine-Glycine: Contains glycine instead of methionine, resulting in different metabolic and physiological effects.
Methionine-Isoleucine: Isoleucine replaces leucine, leading to variations in its role in protein synthesis and muscle metabolism.
Uniqueness
Methionine-Leucine is unique due to the presence of both methionine and leucine, which confer distinct biochemical properties. Methionine’s role in methylation and leucine’s ability to activate the mTOR pathway make this dipeptide particularly interesting for research in muscle metabolism and protein synthesis.
Properties
CAS No. |
14486-16-9 |
---|---|
Molecular Formula |
C11H22N2O3S |
Molecular Weight |
262.37 g/mol |
IUPAC Name |
2-[(2-amino-4-methylsulfanylbutanoyl)amino]-4-methylpentanoic acid |
InChI |
InChI=1S/C11H22N2O3S/c1-7(2)6-9(11(15)16)13-10(14)8(12)4-5-17-3/h7-9H,4-6,12H2,1-3H3,(H,13,14)(H,15,16) |
InChI Key |
PBOUVYGPDSARIS-UHFFFAOYSA-N |
SMILES |
CC(C)CC(C(=O)O)NC(=O)C(CCSC)N |
Isomeric SMILES |
CC(C)C[C@@H](C(=O)[O-])NC(=O)[C@H](CCSC)[NH3+] |
Canonical SMILES |
CC(C)CC(C(=O)O)NC(=O)C(CCSC)N |
physical_description |
Solid |
Origin of Product |
United States |
Retrosynthesis Analysis
AI-Powered Synthesis Planning: Our tool employs the Template_relevance Pistachio, Template_relevance Bkms_metabolic, Template_relevance Pistachio_ringbreaker, Template_relevance Reaxys, Template_relevance Reaxys_biocatalysis model, leveraging a vast database of chemical reactions to predict feasible synthetic routes.
One-Step Synthesis Focus: Specifically designed for one-step synthesis, it provides concise and direct routes for your target compounds, streamlining the synthesis process.
Accurate Predictions: Utilizing the extensive PISTACHIO, BKMS_METABOLIC, PISTACHIO_RINGBREAKER, REAXYS, REAXYS_BIOCATALYSIS database, our tool offers high-accuracy predictions, reflecting the latest in chemical research and data.
Strategy Settings
Precursor scoring | Relevance Heuristic |
---|---|
Min. plausibility | 0.01 |
Model | Template_relevance |
Template Set | Pistachio/Bkms_metabolic/Pistachio_ringbreaker/Reaxys/Reaxys_biocatalysis |
Top-N result to add to graph | 6 |
Feasible Synthetic Routes
Q1: How does fMet-Leu-Phe interact with neutrophils?
A1: fMet-Leu-Phe binds to specific receptors on the surface of neutrophils, known as formyl peptide receptors (FPRs). [, , , , , ] This binding initiates a cascade of intracellular signaling events, including calcium mobilization, phosphoinositide breakdown, and activation of protein kinase C. [, , , ]
Q2: What are the downstream effects of fMet-Leu-Phe binding to its receptor?
A2: fMet-Leu-Phe binding triggers several key neutrophil functions essential for immune response:
- Chemotaxis: The directed migration of neutrophils towards the source of the fMet-Leu-Phe gradient. [, , , , , , ]
- Superoxide Generation: The production of reactive oxygen species (ROS) like superoxide anions, contributing to the destruction of pathogens. [, , , , , , ]
- Degranulation: The release of enzymes and antimicrobial peptides from neutrophil granules, enhancing the inflammatory response. [, , , , ]
- Aggregation: The clumping together of neutrophils, potentially aiding in the containment of infection. [, , ]
Q3: What is the molecular formula and weight of fMet-Leu-Phe?
A3: The molecular formula of fMet-Leu-Phe is C21H31N3O5S, and its molecular weight is 425.55 g/mol.
Q4: Is there spectroscopic data available for fMet-Leu-Phe?
A5: While the provided research primarily focuses on the biological activity of fMet-Leu-Phe, studies have utilized techniques like fluorescence spectroscopy to analyze the size of the receptor binding pocket and the interaction of fMet-Leu-Phe analogs with the receptor. [] Further investigation into spectroscopic databases would be required for comprehensive data.
Q5: How do structural modifications of fMet-Leu-Phe affect its activity?
A5: Studies using various fMet-Leu-Phe analogs have revealed crucial structural requirements for receptor binding and activity:
- N-Formyl Group: The N-formyl group is essential for optimal binding and activity. []
- Amino Acid Side Chains: Modifications at the methionine, leucine, and phenylalanine positions can significantly impact receptor binding affinity and downstream signaling. [, ]
- C-Terminus: Changes at the carboxyl terminus of phenylalanine and beyond can influence the specificity and activity of the peptide. []
Q6: Are there any formulation strategies to improve its stability or bioavailability?
A6: While not explicitly discussed in the provided papers, potential formulation strategies for peptides like fMet-Leu-Phe could involve:
Q7: What in vitro models are used to study fMet-Leu-Phe activity?
A9: Researchers commonly use isolated neutrophils, neutrophil-like cell lines (e.g., HL-60 cells), and endothelial cell cultures to investigate the effects of fMet-Leu-Phe on various cellular functions. [, , , , , , ]
Q8: Are there any animal models used to study fMet-Leu-Phe in vivo?
A10: Yes, rabbit models have been employed to study the role of fMet-Leu-Phe in inflammatory responses, particularly in skin and lung tissues. [, , ]
Disclaimer and Information on In-Vitro Research Products
Please be aware that all articles and product information presented on BenchChem are intended solely for informational purposes. The products available for purchase on BenchChem are specifically designed for in-vitro studies, which are conducted outside of living organisms. In-vitro studies, derived from the Latin term "in glass," involve experiments performed in controlled laboratory settings using cells or tissues. It is important to note that these products are not categorized as medicines or drugs, and they have not received approval from the FDA for the prevention, treatment, or cure of any medical condition, ailment, or disease. We must emphasize that any form of bodily introduction of these products into humans or animals is strictly prohibited by law. It is essential to adhere to these guidelines to ensure compliance with legal and ethical standards in research and experimentation.