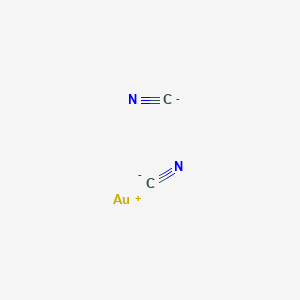
Dicyanoaurate ion
Overview
Description
The dicyanoaurate ion, [Au(CN)₂]⁻, is a linear anion with gold(I) coordinated to two cyanide ligands. It is highly stable, with a formation constant (logβ₂) of 39.3 (β₂ ≈ 2 × 10³⁸) , making it central to gold extraction via cyanidation. Its structure facilitates weak aurophilic (Au–Au) interactions in solution (~3.64 Å in K[Au(CN)₂]), which strengthen under electronic excitation or mechanical stress . Applications span industrial (gold leaching, electroplating) , medical (anti-arthritic drug metabolite) , and materials science (mechanical resilience in zinc dicyanoaurate) .
Preparation Methods
Synthetic Routes and Reaction Conditions
The dicyanoaurate ion can be synthesized by reacting gold(I) chloride with an excess of potassium cyanide: [ \text{AuCl} + 2 \text{KCN} \rightarrow \text{KAu(CN)}_2 + \text{KCl} ]
Industrial Production Methods
Chemical Reactions Analysis
Types of Reactions
The dicyanoaurate ion undergoes various chemical reactions, including:
Oxidation: The ion can be oxidized to form tetracyanoaurate(III) complexes.
Reduction: It can be reduced to metallic gold.
Substitution: The cyanide ligands can be substituted with other ligands, such as halides or phosphines.
Common Reagents and Conditions
Oxidation: Strong oxidizing agents like chlorine or bromine.
Reduction: Reducing agents such as sodium borohydride or hydrazine.
Substitution: Ligand exchange reactions typically occur in the presence of excess ligands and under controlled pH conditions.
Major Products
Oxidation: Tetracyanoaurate(III) complexes.
Reduction: Metallic gold.
Substitution: Various gold(I) complexes with different ligands.
Scientific Research Applications
Synthesis of Gold Nanoparticles
One of the primary applications of dicyanoaurate ions is in the synthesis of gold nanoparticles. These nanoparticles are crucial in various fields, including catalysis, electronics, and biomedicine. The synthesis typically involves the reduction of dicyanoaurate ions using reducing agents such as sodium borohydride or citrate, leading to the formation of stable gold nanoparticles.
Gold Cyanidation Process
Dicyanoaurate ions play a pivotal role in the gold cyanidation process, which is widely used for extracting gold from ores. The mechanism involves the formation of soluble gold complexes that can be easily separated from the ore matrix. This method remains one of the most efficient techniques for gold recovery in mining operations.
Process | Reagents | Outcome |
---|---|---|
Gold extraction | Dicyanoaurate ions | Formation of soluble gold complexes |
Synthesis of nanoparticles | Reducing agents | Formation of gold nanoparticles |
Biological Imaging
Research has indicated potential applications of dicyanoaurate ions in biological imaging. Their ability to form stable complexes with biomolecules allows for enhanced imaging contrast in techniques such as fluorescence microscopy. Studies have shown that dicyanoaurate ions can be used to label specific proteins or cellular components, facilitating detailed imaging studies.
Therapeutic Uses
Dicyanoaurate ions have been investigated for their pharmacological properties, particularly in treating conditions like rheumatoid arthritis. The ion interacts with metal ions and proteins, potentially modulating inflammatory pathways and providing therapeutic benefits.
Gold Plating
In industrial settings, dicyanoaurate ions are extensively used in electroplating processes. The stability and solubility of the ion make it an ideal candidate for depositing gold onto various substrates, enhancing their aesthetic appeal and corrosion resistance.
Material Science
The unique properties of dicyanoaurate ions have led to their exploration in material science. They serve as precursors for creating advanced materials with tailored properties, including conductive polymers and nanocomposites.
Case Study 1: Sorption Kinetics
A study examined the sorption kinetics of dicyanoaurate ions on gold-containing ores from the Vasilkovskoye deposit. The research highlighted how varying concentrations of dicyanoaurate ions influenced the rate of gold extraction through sorption leaching processes, demonstrating the ion's effectiveness in enhancing gold recovery rates .
Case Study 2: Exciplex Formation
Spectroscopic studies revealed that dicyanoaurate ions could form excimers and exciplexes when doped with other materials. This finding has implications for developing new photonic devices that leverage these interactions for enhanced performance .
Mechanism of Action
The dicyanoaurate ion exerts its effects through various mechanisms:
Molecular Targets: It interacts with metal ions and proteins, forming stable complexes.
Pathways Involved: The ion can participate in electron transfer reactions and coordinate with biological molecules, affecting their function and activity
Comparison with Similar Compounds
Comparison with Other Dicyanometallate(I) Ions
Dicyanoargentate(I) ([Ag(CN)₂]⁻)
- Stability : [Ag(CN)₂]⁻ has a lower formation constant (logβ₂ ≈ 20.5) compared to [Au(CN)₂]⁻, reflecting weaker metal-ligand bonds .
- Oligomerization : Both ions aggregate in solution via metal-metal interactions, but [Au(CN)₂]⁻ exhibits stronger aurophilicity (absorption red shift: 13.4 × 10³ cm⁻¹ for Au vs. 11.9 × 10³ cm⁻¹ for Ag at saturation) .
- Luminescence : [Ag(CN)₂]⁻ lacks the distinct luminescence bands seen in [Au(CN)₂]⁻ (e.g., 390 nm and 630 nm in K[Au(CN)₂]) due to weaker metallophilic interactions .
- Selectivity : Macrocyclic hosts like bambusuril bind [Au(CN)₂]⁻ with micromolar affinity in water, outperforming [Ag(CN)₂]⁻ due to stronger chaotropic effects .
Dicyanocuprate(I) ([Cu(CN)₂]⁻)
- Stability : [Cu(CN)₂]⁻ is less stable (logβ₂ ≈ 16) and prone to oxidation, limiting its industrial utility .
Comparison with Other Cyanometallates
Hexacyanoferrate(III) ([Fe(CN)₆]³⁻)
- Bonding : Iron(III) forms octahedral complexes with stronger ionic bonding, contrasting with the covalent Au–CN bonds in [Au(CN)₂]⁻ .
- Applications : [Fe(CN)₆]³⁻ is used in electrochemistry and pigments, whereas [Au(CN)₂]⁻ specializes in gold recovery and functional materials .
Tetracyanopalladate(II) ([Pd(CN)₄]²⁻)
- Geometry : Square-planar Pd(II) complexes lack the linearity and aurophilic interactions of [Au(CN)₂]⁻.
- Luminescence : Pd(II) cyanides exhibit ligand-centered emissions, unlike the metal-centric luminescence of [Au(CN)₂]⁻ .
Stability and Bonding Analysis
Compound | Formation Constant (logβ₂) | Bond Type | Key Interaction |
---|---|---|---|
[Au(CN)₂]⁻ | 39.3 | Covalent | Aurophilic (Au–Au) |
[Ag(CN)₂]⁻ | ~20.5 | Ionic | Argentophilic (Ag–Ag) |
[Cu(CN)₂]⁻ | ~16 | Ionic | None significant |
The exceptional stability of [Au(CN)₂]⁻ arises from relativistic effects strengthening Au–CN bonds and Au–Au interactions (3.10–3.64 Å) .
Luminescence and Aurophilic Interactions
- Emission Bands: K[Au(CN)₂]: 390 nm (aurophilic) and 630 nm (ligand-centered) . [C₅H₁₀NH₂][Au(CN)₂]: 436 nm (shorter Au–Au: 3.10 Å) . Zinc dicyanoaurate: 442 nm (helical supramolecular structure) .
- Mechanism: Excitation induces structural changes (bent → linear oligomers), enhancing covalent Au–Au bonding .
Mechanical Properties in Coordination Polymers
Zinc dicyanoaurate exhibits "giant" elastic recovery (We ≈ 0.85) due to helical Au–CN–Zn motifs acting as atomic springs . Comparable frameworks (e.g., ZIF-8) lack such resilience, with We < 0.5 .
Biological Activity
The dicyanoaurate ion, represented as , is a complex of gold that has garnered attention for its diverse biological activities. This article explores its biological implications, including its effects on inflammation, cellular mechanisms, and potential therapeutic applications.
Dicyanoaurate ions are known for their high chemical stability , which facilitates their use in various applications, including gold processing and electroplating. The ion is also a metabolite derived from gold-based drugs, such as auranofin, which are used in treating autoimmune diseases. Its stability is attributed to the strong bonding between gold and cyanide ions, making it a significant compound in both biological and industrial contexts .
Immune Modulation
Dicyanoaurate ions exhibit immunosuppressive properties , particularly in the context of autoimmune diseases. Studies have shown that these ions can influence lymphokine production, which plays a crucial role in immune responses. For instance, they reduce the activity of macrophages, which are vital for inflammation and tissue repair. This effect is mediated through the binding of dicyanoaurate to proteins containing sulfur within the cells, leading to alterations in cytokine production and reduced collagen synthesis .
Interaction with Cellular Channels
Research has demonstrated that dicyanoaurate ions inhibit the cystic fibrosis transmembrane conductance regulator (CFTR) chloride channels. At low concentrations, these ions decrease the open probability of CFTR channels, while higher concentrations block channel currents by physically occluding the pore. This dual mechanism suggests that dicyanoaurate may interfere with cellular ion transport processes, potentially leading to broader implications for cellular physiology .
Autoimmune Diseases
Dicyanoaurate ions have been utilized in therapies for conditions such as rheumatoid arthritis. They are believed to exert beneficial effects by modulating immune responses and reducing inflammation. The long-term administration of gold compounds containing dicyanoaurate has been shown to lead to significant clinical improvements in patients with autoimmune disorders .
Antimicrobial Activity
Emerging studies indicate that dicyanoaurate may possess antimicrobial properties. While specific mechanisms are still under investigation, its interactions with microbial membranes could disrupt cellular integrity, leading to bactericidal effects. This potential application is particularly relevant given the increasing resistance to conventional antibiotics .
Case Studies and Research Findings
Study | Focus | Findings |
---|---|---|
Tepperman et al. (1994) | Autoimmune Inflammation | Dicyanoaurate ions reduce macrophage activity and collagen production in treated patients. |
Balfourier et al. (2020) | Rheumatoid Arthritis | Gold complexes show temporal benefits on inflammation markers in clinical settings. |
Linsdell et al. (2001) | CFTR Channel Inhibition | Demonstrated dual mechanisms of inhibition by dicyanoaurate on CFTR channels, affecting ion transport dynamics. |
Q & A
Q. Basic: What experimental methods are recommended for synthesizing and characterizing dicyanoaurate ions in aqueous solutions?
Methodological Answer:
The dicyanoaurate ion ([Au(CN)₂]⁻) is typically synthesized via cyanide leaching processes under alkaline and oxygenated conditions. Key steps include:
- Synthesis : Reacting gold with cyanide salts (e.g., KCN or NaCN) in an aqueous alkaline medium (pH > 10) with aeration to ensure oxygen availability, as described by the Elsner equation:
. - Characterization : UV-vis spectroscopy (absorption at ~540 nm for gold sol intermediates) , Raman spectroscopy (C≡N stretching modes ~2100 cm⁻¹), and X-ray crystallography to confirm linear geometry (bond angle ~180°) .
Q. Advanced: How can diffusion mechanisms in this compound sorption be experimentally distinguished?
Methodological Answer:
To differentiate between external and internal diffusion limitations in sorption kinetics (e.g., using anion-exchange resins):
- Variable Concentration Studies : Plot sorption rate () against initial gold concentration. A linear relationship () suggests external diffusion dominance, while deviations indicate intra-particle diffusion .
- Temperature-Dependent Analysis : Calculate activation energy () via the Arrhenius equation. kJ/mol implies diffusion control, whereas higher values suggest chemical reaction control .
- Model Fitting : Use the Weber-Morris intra-particle diffusion model () and Boyd’s film diffusion model. Mixed diffusion is confirmed if both models partially fit experimental data .
Q. Advanced: What structural analysis techniques resolve the coordination behavior of dicyanoaurate in supramolecular networks?
Methodological Answer:
- X-ray Absorption Spectroscopy (XAS) : Probe Au L₃-edge spectra to determine oxidation state (Au⁺) and local coordination geometry. EXAFS fitting reveals Au-C/N bond distances (~2.0 Å) .
- Single-Crystal XRD : Analyze intermolecular interactions (e.g., aurophilic Au···Au contacts < 3.5 Å) and hydrogen bonding (e.g., C≡N···H distances ~2.2 Å) in frameworks like Zn-dicyanoaurate .
- Solid-State NMR : ¹³C NMR detects cyanide ligand environments, while ¹H NMR identifies hydrogen-bonding networks in hydrated phases .
Q. Basic: How can spectrophotometry quantify dicyanoaurate ions in environmental or biological systems?
Methodological Answer:
- Gold Sol Displacement Method : Measure dissolved oxygen (DO) by monitoring absorbance decay at 540 nm as gold sol (Au⁰ nanoparticles) oxidizes to [Au(CN)₂]⁻ under cyanide excess. Calibration curves (DO vs. ΔA) achieve detection limits of ~0.1 mg/L .
- Interference Mitigation : Pre-treat samples with EDTA to chelate competing metal ions (e.g., Cu²⁺, Fe³⁺) that may bind cyanide .
Q. Advanced: How do researchers resolve contradictions in reported optimal temperatures for dicyanoaurate sorption?
Methodological Answer:
Discrepancies in optimal sorption temperatures (e.g., 298 K vs. 308–318 K) arise from differences in:
- Cyanide/Alkalinity Levels : Higher CN⁻/OH⁻ concentrations stabilize [Au(CN)₂]⁻ at elevated temperatures, while low-cyanide systems favor sorption at 298 K .
- Resin Porosity : Macroporous resins reduce intra-diffusion barriers, shifting optimal temperatures upward. Validate via BET surface area analysis and pore-size distribution .
- Competing Ions : Presence of Cu(CN)₃²⁻ or Ag(CN)₂⁻ alters activation energy requirements. Use ICP-MS to quantify co-adsorbed species .
Q. Basic: What safety protocols are critical when handling potassium dicyanoaurate in laboratory settings?
Methodological Answer:
- Exposure Limits : Adhere to DNEL thresholds:
- Inhalation: ≤0.071 mg/m³ (long-term)
- Dermal: ≤0.1 mg/kg/day .
- Waste Management : Neutralize cyanide waste with H₂O₂ (pH 10–12) to convert CN⁻ to OCN⁻, followed by Fe²⁺ precipitation .
- PPE : Use nitrile gloves, fume hoods, and respiratory protection during synthesis .
Q. Advanced: How does dicyanoaurate’s supramolecular behavior enable novel material properties?
Methodological Answer:
- Negative Linear Compressibility (NLC) : In Zn-dicyanoaurate, Au···Au aurophilic interactions and flexible CN⁻ bridges allow spring-like helix compression under pressure (ΔV/ΔP < 0), applicable in pressure sensors .
- Luminescence : [Au(CN)₂]⁻ aggregates exhibit tunable emission via cation-π interactions (e.g., K⁺ vs. Rb⁺ doping shifts λₑₘ from 450 to 520 nm) .
- Self-Assembly : In solution, oligomerization precedes crystallization. Monitor via ESI-MS (m/z peaks for [Au₃Zn₂(CN)₆]ⁿ⁻ clusters) and UV-vis (absorbance shifts ~300 nm) .
Properties
IUPAC Name |
gold(1+);dicyanide | |
---|---|---|
Source | PubChem | |
URL | https://pubchem.ncbi.nlm.nih.gov | |
Description | Data deposited in or computed by PubChem | |
InChI |
InChI=1S/2CN.Au/c2*1-2;/q2*-1;+1 | |
Source | PubChem | |
URL | https://pubchem.ncbi.nlm.nih.gov | |
Description | Data deposited in or computed by PubChem | |
InChI Key |
QGIPNVHDSSGMAA-UHFFFAOYSA-N | |
Source | PubChem | |
URL | https://pubchem.ncbi.nlm.nih.gov | |
Description | Data deposited in or computed by PubChem | |
Canonical SMILES |
[C-]#N.[C-]#N.[Au+] | |
Source | PubChem | |
URL | https://pubchem.ncbi.nlm.nih.gov | |
Description | Data deposited in or computed by PubChem | |
Molecular Formula |
C2AuN2- | |
Source | PubChem | |
URL | https://pubchem.ncbi.nlm.nih.gov | |
Description | Data deposited in or computed by PubChem | |
Related CAS |
13967-50-5 (potassium salt), 15280-09-8 (hydrochloride salt), 31096-40-9 (ammonium salt) | |
Record name | Dicyanoaurate | |
Source | ChemIDplus | |
URL | https://pubchem.ncbi.nlm.nih.gov/substance/?source=chemidplus&sourceid=0014950879 | |
Description | ChemIDplus is a free, web search system that provides access to the structure and nomenclature authority files used for the identification of chemical substances cited in National Library of Medicine (NLM) databases, including the TOXNET system. | |
Molecular Weight |
249.00 g/mol | |
Source | PubChem | |
URL | https://pubchem.ncbi.nlm.nih.gov | |
Description | Data deposited in or computed by PubChem | |
CAS No. |
14950-87-9 | |
Record name | Dicyanoaurate(1-) | |
Source | CAS Common Chemistry | |
URL | https://commonchemistry.cas.org/detail?cas_rn=14950-87-9 | |
Description | CAS Common Chemistry is an open community resource for accessing chemical information. Nearly 500,000 chemical substances from CAS REGISTRY cover areas of community interest, including common and frequently regulated chemicals, and those relevant to high school and undergraduate chemistry classes. This chemical information, curated by our expert scientists, is provided in alignment with our mission as a division of the American Chemical Society. | |
Explanation | The data from CAS Common Chemistry is provided under a CC-BY-NC 4.0 license, unless otherwise stated. | |
Record name | Dicyanoaurate | |
Source | ChemIDplus | |
URL | https://pubchem.ncbi.nlm.nih.gov/substance/?source=chemidplus&sourceid=0014950879 | |
Description | ChemIDplus is a free, web search system that provides access to the structure and nomenclature authority files used for the identification of chemical substances cited in National Library of Medicine (NLM) databases, including the TOXNET system. | |
Record name | DICYANOAURATE ION | |
Source | FDA Global Substance Registration System (GSRS) | |
URL | https://gsrs.ncats.nih.gov/ginas/app/beta/substances/I0XSL5544A | |
Description | The FDA Global Substance Registration System (GSRS) enables the efficient and accurate exchange of information on what substances are in regulated products. Instead of relying on names, which vary across regulatory domains, countries, and regions, the GSRS knowledge base makes it possible for substances to be defined by standardized, scientific descriptions. | |
Explanation | Unless otherwise noted, the contents of the FDA website (www.fda.gov), both text and graphics, are not copyrighted. They are in the public domain and may be republished, reprinted and otherwise used freely by anyone without the need to obtain permission from FDA. Credit to the U.S. Food and Drug Administration as the source is appreciated but not required. | |
Retrosynthesis Analysis
AI-Powered Synthesis Planning: Our tool employs the Template_relevance Pistachio, Template_relevance Bkms_metabolic, Template_relevance Pistachio_ringbreaker, Template_relevance Reaxys, Template_relevance Reaxys_biocatalysis model, leveraging a vast database of chemical reactions to predict feasible synthetic routes.
One-Step Synthesis Focus: Specifically designed for one-step synthesis, it provides concise and direct routes for your target compounds, streamlining the synthesis process.
Accurate Predictions: Utilizing the extensive PISTACHIO, BKMS_METABOLIC, PISTACHIO_RINGBREAKER, REAXYS, REAXYS_BIOCATALYSIS database, our tool offers high-accuracy predictions, reflecting the latest in chemical research and data.
Strategy Settings
Precursor scoring | Relevance Heuristic |
---|---|
Min. plausibility | 0.01 |
Model | Template_relevance |
Template Set | Pistachio/Bkms_metabolic/Pistachio_ringbreaker/Reaxys/Reaxys_biocatalysis |
Top-N result to add to graph | 6 |
Feasible Synthetic Routes
Disclaimer and Information on In-Vitro Research Products
Please be aware that all articles and product information presented on BenchChem are intended solely for informational purposes. The products available for purchase on BenchChem are specifically designed for in-vitro studies, which are conducted outside of living organisms. In-vitro studies, derived from the Latin term "in glass," involve experiments performed in controlled laboratory settings using cells or tissues. It is important to note that these products are not categorized as medicines or drugs, and they have not received approval from the FDA for the prevention, treatment, or cure of any medical condition, ailment, or disease. We must emphasize that any form of bodily introduction of these products into humans or animals is strictly prohibited by law. It is essential to adhere to these guidelines to ensure compliance with legal and ethical standards in research and experimentation.