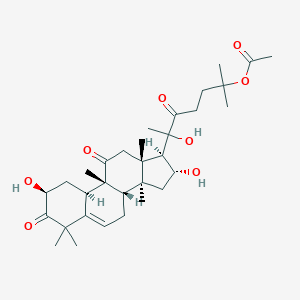
Dihydrocucurbitacin B
Overview
Description
Dihydrocucurbitacin B is a naturally occurring compound belonging to the cucurbitacin family, which are highly oxidized tetracyclic triterpenoids. These compounds are primarily found in the Cucurbitaceae family of plants, but they are also present in other plant families, fungi, and some marine organisms . This compound has garnered significant attention due to its potent biological activities, including anti-inflammatory, hepatoprotective, and anticancer properties .
Mechanism of Action
Target of Action
Dihydrocucurbitacin B, a triterpenoid isolated from the plant Hemsleya amabilis, primarily targets the T-cell nuclear factor . It also targets the protein GRP78, which is involved in the GRP78/FOXM1/KIF20A signaling pathway .
Mode of Action
This compound inhibits the activation of the T-cell nuclear factor, leading to cell cycle arrest at the G0 phase . It also suppresses delayed-type hypersensitivity reactions . By targeting the protein GRP78, it downregulates the GRP78/FOXM1/KIF20A signaling pathway .
Biochemical Pathways
This compound affects several biochemical pathways. It induces apoptosis and suppresses cell migration and invasion . It also inhibits cell growth and survival, and has anti-inflammatory effects .
Pharmacokinetics
The pharmacokinetics of cucurbitacins, including this compound, involve absorption, metabolism, distribution, and elimination . These compounds have a strong affinity for albumin binding without causing significant structural changes in the HSA protein .
Result of Action
The molecular and cellular effects of this compound’s action include the induction of apoptosis, suppression of cell migration and invasion, cell cycle arrest, inhibition of cell growth and survival, and anti-inflammatory effects .
Biochemical Analysis
Biochemical Properties
Dihydrocucurbitacin B has been reported to interact with a variety of recognized cellular targets to impede the growth of cancer cells . It has been found to inhibit cell proliferation without significant effects on cell viability . The compound has been shown to interact with enzymes such as elastase, cyclooxygenase-2, and nitric oxide synthase-2 .
Cellular Effects
This compound has significant effects on various types of cells and cellular processes. It has been shown to reduce cell proliferation due to a decrease in the expression of cyclins, mainly cyclin-B1, and disruption of the actin cytoskeleton, arresting B16F10 cells in G2/M phase . It also has been reported to have anti-inflammatory effects in mice .
Molecular Mechanism
The molecular mechanism of this compound involves interactions with numerous cellular targets. It has been shown to increase SREBP2 protein levels and decrease HNF1 protein levels in the nuclei . This dual transcriptional regulation of LDLR and PCSK9 in HepG2 cells leads to an increase in the expression of LDLR protein and a decrease in PCSK9-mediated LDLR protein degradation .
Temporal Effects in Laboratory Settings
In laboratory settings, this compound has been shown to dose-dependently promote DiI-LDL uptake in HepG2 cells by upregulating low-density lipoprotein receptor (LDLR) protein . This suggests that the effects of this compound can change over time in laboratory settings.
Dosage Effects in Animal Models
In a high-cholesterol LVG golden Syrian Hamster model, administration of this compound (30 mg·kg−1·d−1, intragastric, for 3 weeks) significantly decreased the serum LDL-cholesterol (LDL-C) levels . PCSK9 protein levels in the serum and liver tissues were significantly decreased, whereas LDLR protein levels in liver tissues were significantly increased .
Metabolic Pathways
This compound is involved in the cholesterol synthesis pathway. Depletion of intracellular cholesterol activates the proteolytic cleavage of sterol regulatory element binding protein 2 (SREBP2) and releases the mature form of SREBP2 into the cell nucleus .
Transport and Distribution
This compound has been shown to promote DiI-LDL uptake in HepG2 cells by upregulating LDLR protein . This suggests that this compound may be transported and distributed within cells and tissues via LDLR protein.
Subcellular Localization
Given its role in upregulating LDLR protein and its involvement in the cholesterol synthesis pathway, it can be inferred that it may be localized in the cell nucleus .
Preparation Methods
Synthetic Routes and Reaction Conditions: The synthesis of dihydrocucurbitacin B involves several steps, starting from simpler triterpenoid precursors. One common synthetic route includes the oxidation of cucurbitacin B to form this compound. This process typically involves the use of strong oxidizing agents such as potassium permanganate or chromium trioxide under controlled conditions .
Industrial Production Methods: Industrial production of this compound often relies on extraction from natural sources, such as the roots of Siraitia grosvenorii. High-speed countercurrent chromatography (HSCCC) is a widely used technique for the separation and purification of cucurbitacins from plant extracts. This method involves the use of a solvent system, such as n-hexane-ethyl acetate-methanol-water, to partition the cucurbitacin fraction and remove impurities .
Chemical Reactions Analysis
Types of Reactions: Dihydrocucurbitacin B undergoes various chemical reactions, including:
Oxidation: Conversion to more oxidized forms using agents like potassium permanganate.
Reduction: Reduction to less oxidized forms using reducing agents such as sodium borohydride.
Substitution: Introduction of different functional groups through reactions with reagents like succinic anhydride.
Common Reagents and Conditions:
Oxidation: Potassium permanganate in an aqueous medium.
Reduction: Sodium borohydride in tetrahydrofuran (THF).
Substitution: Succinic anhydride in the presence of dimethylaminopyridine (DMAP) and dichloromethane (DCM).
Major Products:
Oxidation: More oxidized cucurbitacin derivatives.
Reduction: Less oxidized cucurbitacin derivatives.
Substitution: Succinylated cucurbitacin derivatives.
Scientific Research Applications
Dihydrocucurbitacin B has a wide range of scientific research applications:
Biology: Studied for its role in plant defense mechanisms against herbivores and pathogens.
Medicine: Investigated for its anticancer, anti-inflammatory, and hepatoprotective activities.
Industry: Utilized in the development of natural pesticides and as a lead compound for drug development.
Comparison with Similar Compounds
Dihydrocucurbitacin B is unique among cucurbitacins due to its specific structural features and biological activities. Similar compounds include:
Cucurbitacin B: Shares similar anticancer and anti-inflammatory properties but differs in its oxidation state.
Cucurbitacin E: Known for its potent anticancer activity but has a different acetylation pattern.
Dihydrocucurbitacin E: Similar to this compound but with variations in its functional groups.
This compound stands out due to its balanced profile of biological activities and relatively lower toxicity compared to some other cucurbitacins .
Properties
IUPAC Name |
[(6R)-6-[(2S,8S,9R,10R,13R,14S,16R,17R)-2,16-dihydroxy-4,4,9,13,14-pentamethyl-3,11-dioxo-2,7,8,10,12,15,16,17-octahydro-1H-cyclopenta[a]phenanthren-17-yl]-6-hydroxy-2-methyl-5-oxoheptan-2-yl] acetate | |
---|---|---|
Source | PubChem | |
URL | https://pubchem.ncbi.nlm.nih.gov | |
Description | Data deposited in or computed by PubChem | |
InChI |
InChI=1S/C32H48O8/c1-17(33)40-27(2,3)13-12-23(36)32(9,39)25-21(35)15-29(6)22-11-10-18-19(14-20(34)26(38)28(18,4)5)31(22,8)24(37)16-30(25,29)7/h10,19-22,25,34-35,39H,11-16H2,1-9H3/t19-,20+,21-,22+,25+,29+,30-,31+,32+/m1/s1 | |
Source | PubChem | |
URL | https://pubchem.ncbi.nlm.nih.gov | |
Description | Data deposited in or computed by PubChem | |
InChI Key |
QZJJDOYZVRUEDY-NRNCYQGDSA-N | |
Source | PubChem | |
URL | https://pubchem.ncbi.nlm.nih.gov | |
Description | Data deposited in or computed by PubChem | |
Canonical SMILES |
CC(=O)OC(C)(C)CCC(=O)C(C)(C1C(CC2(C1(CC(=O)C3(C2CC=C4C3CC(C(=O)C4(C)C)O)C)C)C)O)O | |
Source | PubChem | |
URL | https://pubchem.ncbi.nlm.nih.gov | |
Description | Data deposited in or computed by PubChem | |
Isomeric SMILES |
CC(=O)OC(C)(C)CCC(=O)[C@@](C)([C@H]1[C@@H](C[C@@]2([C@@]1(CC(=O)[C@@]3([C@H]2CC=C4[C@H]3C[C@@H](C(=O)C4(C)C)O)C)C)C)O)O | |
Source | PubChem | |
URL | https://pubchem.ncbi.nlm.nih.gov | |
Description | Data deposited in or computed by PubChem | |
Molecular Formula |
C32H48O8 | |
Source | PubChem | |
URL | https://pubchem.ncbi.nlm.nih.gov | |
Description | Data deposited in or computed by PubChem | |
DSSTOX Substance ID |
DTXSID701314710 | |
Record name | Dihydrocucurbitacin B | |
Source | EPA DSSTox | |
URL | https://comptox.epa.gov/dashboard/DTXSID701314710 | |
Description | DSSTox provides a high quality public chemistry resource for supporting improved predictive toxicology. | |
Molecular Weight |
560.7 g/mol | |
Source | PubChem | |
URL | https://pubchem.ncbi.nlm.nih.gov | |
Description | Data deposited in or computed by PubChem | |
CAS No. |
13201-14-4 | |
Record name | Dihydrocucurbitacin B | |
Source | CAS Common Chemistry | |
URL | https://commonchemistry.cas.org/detail?cas_rn=13201-14-4 | |
Description | CAS Common Chemistry is an open community resource for accessing chemical information. Nearly 500,000 chemical substances from CAS REGISTRY cover areas of community interest, including common and frequently regulated chemicals, and those relevant to high school and undergraduate chemistry classes. This chemical information, curated by our expert scientists, is provided in alignment with our mission as a division of the American Chemical Society. | |
Explanation | The data from CAS Common Chemistry is provided under a CC-BY-NC 4.0 license, unless otherwise stated. | |
Record name | DIHYDROCUCURBITACIN B | |
Source | DTP/NCI | |
URL | https://dtp.cancer.gov/dtpstandard/servlet/dwindex?searchtype=NSC&outputformat=html&searchlist=106401 | |
Description | The NCI Development Therapeutics Program (DTP) provides services and resources to the academic and private-sector research communities worldwide to facilitate the discovery and development of new cancer therapeutic agents. | |
Explanation | Unless otherwise indicated, all text within NCI products is free of copyright and may be reused without our permission. Credit the National Cancer Institute as the source. | |
Record name | Dihydrocucurbitacin B | |
Source | EPA DSSTox | |
URL | https://comptox.epa.gov/dashboard/DTXSID701314710 | |
Description | DSSTox provides a high quality public chemistry resource for supporting improved predictive toxicology. | |
Record name | DIHYDROCUCURBITACIN B | |
Source | FDA Global Substance Registration System (GSRS) | |
URL | https://gsrs.ncats.nih.gov/ginas/app/beta/substances/ZYL5IKR83R | |
Description | The FDA Global Substance Registration System (GSRS) enables the efficient and accurate exchange of information on what substances are in regulated products. Instead of relying on names, which vary across regulatory domains, countries, and regions, the GSRS knowledge base makes it possible for substances to be defined by standardized, scientific descriptions. | |
Explanation | Unless otherwise noted, the contents of the FDA website (www.fda.gov), both text and graphics, are not copyrighted. They are in the public domain and may be republished, reprinted and otherwise used freely by anyone without the need to obtain permission from FDA. Credit to the U.S. Food and Drug Administration as the source is appreciated but not required. | |
Retrosynthesis Analysis
AI-Powered Synthesis Planning: Our tool employs the Template_relevance Pistachio, Template_relevance Bkms_metabolic, Template_relevance Pistachio_ringbreaker, Template_relevance Reaxys, Template_relevance Reaxys_biocatalysis model, leveraging a vast database of chemical reactions to predict feasible synthetic routes.
One-Step Synthesis Focus: Specifically designed for one-step synthesis, it provides concise and direct routes for your target compounds, streamlining the synthesis process.
Accurate Predictions: Utilizing the extensive PISTACHIO, BKMS_METABOLIC, PISTACHIO_RINGBREAKER, REAXYS, REAXYS_BIOCATALYSIS database, our tool offers high-accuracy predictions, reflecting the latest in chemical research and data.
Strategy Settings
Precursor scoring | Relevance Heuristic |
---|---|
Min. plausibility | 0.01 |
Model | Template_relevance |
Template Set | Pistachio/Bkms_metabolic/Pistachio_ringbreaker/Reaxys/Reaxys_biocatalysis |
Top-N result to add to graph | 6 |
Feasible Synthetic Routes
Disclaimer and Information on In-Vitro Research Products
Please be aware that all articles and product information presented on BenchChem are intended solely for informational purposes. The products available for purchase on BenchChem are specifically designed for in-vitro studies, which are conducted outside of living organisms. In-vitro studies, derived from the Latin term "in glass," involve experiments performed in controlled laboratory settings using cells or tissues. It is important to note that these products are not categorized as medicines or drugs, and they have not received approval from the FDA for the prevention, treatment, or cure of any medical condition, ailment, or disease. We must emphasize that any form of bodily introduction of these products into humans or animals is strictly prohibited by law. It is essential to adhere to these guidelines to ensure compliance with legal and ethical standards in research and experimentation.