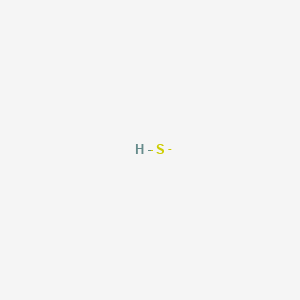
Hydrosulfide
Overview
Description
Hydrosulfide, also known as hydrogen sulfide, is a chemical compound with the formula H₂S. It is a colorless gas with a characteristic foul odor of rotten eggs. This compound is naturally occurring and can be found in volcanic gases, natural gas, and as a byproduct of the decomposition of organic matter. Hydrogen sulfide is highly toxic and flammable, making it a compound of significant interest in both scientific and industrial contexts .
Preparation Methods
Synthetic Routes and Reaction Conditions: Hydrogen sulfide can be synthesized in the laboratory by treating ferrous sulfide with a strong acid, such as hydrochloric acid, in a Kipp generator. The reaction is as follows:
FeS+2HCl→FeCl2+H2S
Another method involves the use of thioacetamide, which generates hydrogen sulfide upon hydrolysis:
CH3C(S)NH2+H2O→CH3C(O)NH2+H2S
Properties
CAS No. |
15035-72-0 |
---|---|
Molecular Formula |
HS- |
Molecular Weight |
33.08 g/mol |
IUPAC Name |
sulfanide |
InChI |
InChI=1S/H2S/h1H2/p-1 |
InChI Key |
RWSOTUBLDIXVET-UHFFFAOYSA-M |
SMILES |
[SH-] |
Canonical SMILES |
[SH-] |
Synonyms |
Sulfide (hs1-) |
Origin of Product |
United States |
Synthesis routes and methods
Procedure details
Disclaimer and Information on In-Vitro Research Products
Please be aware that all articles and product information presented on BenchChem are intended solely for informational purposes. The products available for purchase on BenchChem are specifically designed for in-vitro studies, which are conducted outside of living organisms. In-vitro studies, derived from the Latin term "in glass," involve experiments performed in controlled laboratory settings using cells or tissues. It is important to note that these products are not categorized as medicines or drugs, and they have not received approval from the FDA for the prevention, treatment, or cure of any medical condition, ailment, or disease. We must emphasize that any form of bodily introduction of these products into humans or animals is strictly prohibited by law. It is essential to adhere to these guidelines to ensure compliance with legal and ethical standards in research and experimentation.