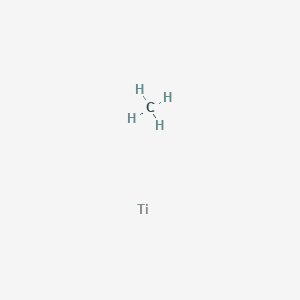
Titanium carbide (TiC)
Overview
Description
Titanium carbide is a refractory ceramic material with the chemical formula TiC. It is known for its extreme hardness, ranking 9-9.5 on the Mohs scale, and has a high melting point of approximately 3,160°C. Titanium carbide appears as a black powder and has a face-centered cubic crystal structure similar to that of sodium chloride. It is found in nature as the rare mineral khamrabaevite, which contains titanium, vanadium, and iron carbides .
Biochemical Analysis
Biochemical Properties
Titanium carbide is known for its high melting point, elastic modulus, high Vickers hardness, low density, high flexure strength, good thermal conductivity, high resistance to corrosion and oxidation, and high thermal shock resistance . There is limited information available on the role of titanium carbide in biochemical reactions and its interactions with enzymes, proteins, and other biomolecules.
Molecular Mechanism
It’s known that titanium carbide has an elastic modulus of approximately 400 GPa and a shear modulus of 188 GPa , which could potentially influence its interactions with biomolecules.
Temporal Effects in Laboratory Settings
The carburisation rate increased from 18.37% to 36.09% for 2 h–10 h of holding time at 1400 °C, and from 51.43% to 77.57% for 2 h–10 h of holding time at 1500 °C .
Metabolic Pathways
The synthesis of titanium carbide involves the main reduction path of TiO2–Magnéli–Ti3O5–Ti2O3–TiCxO1−x .
Subcellular Localization
The subcellular localization of titanium carbide is not well-understood. It’s known that titanium carbide, with chemical formula TiC, crystallizes in the cubic system: NaCl type with space group Fm3m (Z = 4). The lattice constant is a = 0.4327 nm. Ti atoms occupy the origin positions (0,0,0), however, C atoms are located in (1/2,1/2,1/2) positions .
Preparation Methods
Synthetic Routes and Reaction Conditions: Titanium carbide can be synthesized through various methods, including:
Carbothermic Reduction: This involves the reduction of titanium dioxide (TiO₂) with carbon at high temperatures.
Chemical Vapor Deposition (CVD): This method involves the reaction of titanium tetrachloride (TiCl₄) with methane (CH₄) in a hydrogen atmosphere at high temperatures to produce titanium carbide.
Self-Propagating High-Temperature Synthesis (SHS): This method involves the exothermic reaction of titanium and carbon powders, which propagates through the reactant mixture, producing titanium carbide.
Industrial Production Methods: Industrial production of titanium carbide often employs carbothermic reduction due to its cost-effectiveness and scalability. The process involves mixing titanium dioxide with carbon black and heating the mixture in an inert atmosphere to produce titanium carbide .
Chemical Reactions Analysis
Titanium carbide undergoes various chemical reactions, including:
Oxidation: Titanium carbide can be oxidized to titanium dioxide (TiO₂) at high temperatures in the presence of oxygen. The reaction is as follows: [ \text{TiC} + 2\text{O}_2 \rightarrow \text{TiO}_2 + \text{CO}_2 ]
Hydrolysis: Titanium carbide reacts with water to produce methane (CH₄) and titanium dioxide. The reaction is: [ \text{TiC} + x\text{H}_2\text{O} \rightarrow \text{CH}_4 + \text{TiO}_2 \cdot x\text{H}_2\text{O} ]
Reduction: Titanium carbide can be reduced to titanium metal in the presence of a strong reducing agent such as magnesium or calcium.
Common reagents used in these reactions include oxygen, water, and reducing agents like magnesium and calcium. The major products formed from these reactions are titanium dioxide and methane .
Scientific Research Applications
Titanium carbide has a wide range of scientific research applications, including:
Cutting Tools: Titanium carbide is used in the manufacture of cutting tools and abrasives due to its extreme hardness and wear resistance.
Electronics: Titanium carbide is used in electronic devices for its good electrical conductivity and chemical stability.
Composites: Titanium carbide is used as a reinforcing agent in metal matrix composites to improve their mechanical properties.
Environmental Remediation: Titanium carbide serves as an adsorbent for gases such as ammonia, methane, and carbon monoxide due to its large specific surface area and active sites.
Mechanism of Action
The mechanism by which titanium carbide exerts its effects is primarily through its physical properties. The high hardness and melting point of titanium carbide make it an effective material for cutting tools and high-temperature applications. The chemical stability and electrical conductivity of titanium carbide make it suitable for use in electronic devices.
Comparison with Similar Compounds
Titanium carbide is similar to other metal carbides such as tungsten carbide (WC) and silicon carbide (SiC). it has unique properties that distinguish it from these compounds:
Tungsten Carbide (WC): Tungsten carbide is harder than titanium carbide, with a Mohs hardness of 8.5-9.
Silicon Carbide (SiC): Silicon carbide has similar hardness and thermal conductivity to titanium carbide but is more chemically stable and has better oxidation resistance.
Other similar compounds include vanadium carbide (VC) and niobium carbide (NbC), which also have high hardness and melting points but differ in their specific applications and properties .
Biological Activity
Titanium carbide (TiC) is a ceramic material known for its hardness, thermal stability, and excellent wear resistance. Recent studies have begun to explore its biological activity, particularly in biomedical applications such as implants and cancer treatment. This article reviews the biological activity of TiC, focusing on its interactions with biological systems, potential therapeutic applications, and biocompatibility.
1. Overview of Titanium Carbide
Chemical Properties:
- Formula: TiC
- Molecular Weight: 59.89 g/mol
- Structure: Face-centered cubic lattice
TiC is synthesized through various methods including chemical vapor deposition (CVD), plasma spraying, and solid-state reactions. Its unique properties make it suitable for applications in both industrial and biomedical fields.
2. Biocompatibility and Osseointegration
TiC has been investigated for its biocompatibility when used as a coating material for titanium implants. Studies indicate that TiC coatings enhance the biological response of implants:
- Cellular Response : TiC-coated surfaces promote osteoblast adhesion and proliferation compared to uncoated titanium surfaces. This is attributed to improved surface stability and hardness, which facilitate better integration with bone tissue .
- Gene Expression : Research has shown upregulation of genes associated with osteoblast differentiation (e.g., alkaline phosphatase, osteocalcin) when cells are cultured on TiC surfaces .
Table 1: Comparison of Biological Responses on TiC vs. Uncoated Titanium
Parameter | TiC-Coated Implants | Uncoated Titanium |
---|---|---|
Cell Adhesion | Increased | Moderate |
Osteoblast Proliferation | Enhanced | Limited |
Bone Density (after 4 weeks) | Higher | Lower |
Gene Expression (osteogenic) | Upregulated | Baseline |
3. Anticancer Properties
Recent studies have highlighted the potential of TiC in cancer therapy, particularly in overcoming drug resistance in non-small cell lung cancer (NSCLC):
- Mechanism of Action : Two-dimensional titanium carbide (2D TiC) has been shown to reverse cisplatin resistance in NSCLC cells by reducing intracellular antioxidant reserves and altering the expression of drug resistance genes such as MDR-1 and LRP . This suggests that TiC can enhance the efficacy of existing chemotherapeutic agents.
- In Vitro and In Vivo Studies : In vitro assays demonstrated that 2D TiC significantly increased cisplatin uptake in resistant cell lines, while in vivo studies confirmed its tumor inhibitory effects without significant toxicity .
Case Study: Reversal of Chemoresistance
A study involving NSCLC models showed that treatment with 2D TiC led to a substantial decrease in tumor size compared to controls treated with cisplatin alone. The combination therapy resulted in:
- Tumor Size Reduction : Up to 50% reduction after two weeks.
- Survival Rates : Improved survival rates in treated groups compared to controls.
4. Cytotoxicity Studies
The cytotoxic effects of TiC have also been evaluated using various cancer cell lines:
- Graphene-Functionalized TiC : A study reported that graphene-functionalized titanium carbide exhibited significant cytotoxicity against several cancer cell lines, indicating its potential as an anticancer agent . The MTT assay confirmed reduced cell viability upon exposure to this composite material.
Table 2: Cytotoxic Effects of Graphene-Functionalized TiC
Cell Line | IC50 Value (µg/mL) | Observations |
---|---|---|
A549 (Lung Cancer) | 25 | Significant cytotoxicity |
HeLa (Cervical) | 30 | Moderate cytotoxicity |
MCF7 (Breast) | 20 | High sensitivity observed |
5. Conclusion
The biological activity of titanium carbide demonstrates promising applications in both orthopedics and oncology. Its ability to enhance osseointegration makes it an excellent candidate for implant coatings, while its potential to reverse drug resistance positions it as a valuable tool in cancer therapy. Ongoing research is essential to fully elucidate the mechanisms underlying these effects and to translate these findings into clinical practice.
Properties
IUPAC Name |
methane;titanium | |
---|---|---|
Source | PubChem | |
URL | https://pubchem.ncbi.nlm.nih.gov | |
Description | Data deposited in or computed by PubChem | |
InChI |
InChI=1S/CH4.Ti/h1H4; | |
Source | PubChem | |
URL | https://pubchem.ncbi.nlm.nih.gov | |
Description | Data deposited in or computed by PubChem | |
InChI Key |
TXKRDMUDKYVBLB-UHFFFAOYSA-N | |
Source | PubChem | |
URL | https://pubchem.ncbi.nlm.nih.gov | |
Description | Data deposited in or computed by PubChem | |
Canonical SMILES |
C.[Ti] | |
Source | PubChem | |
URL | https://pubchem.ncbi.nlm.nih.gov | |
Description | Data deposited in or computed by PubChem | |
Molecular Formula |
CH4Ti | |
Source | PubChem | |
URL | https://pubchem.ncbi.nlm.nih.gov | |
Description | Data deposited in or computed by PubChem | |
DSSTOX Substance ID |
DTXSID60925760 | |
Record name | Methane--titanium (1/1) | |
Source | EPA DSSTox | |
URL | https://comptox.epa.gov/dashboard/DTXSID60925760 | |
Description | DSSTox provides a high quality public chemistry resource for supporting improved predictive toxicology. | |
Molecular Weight |
63.910 g/mol | |
Source | PubChem | |
URL | https://pubchem.ncbi.nlm.nih.gov | |
Description | Data deposited in or computed by PubChem | |
CAS No. |
12070-08-5, 12700-20-8 | |
Record name | Titanium carbide | |
Source | ChemIDplus | |
URL | https://pubchem.ncbi.nlm.nih.gov/substance/?source=chemidplus&sourceid=0012070085 | |
Description | ChemIDplus is a free, web search system that provides access to the structure and nomenclature authority files used for the identification of chemical substances cited in National Library of Medicine (NLM) databases, including the TOXNET system. | |
Record name | Titanium carbide (TiC) | |
Source | EPA Chemicals under the TSCA | |
URL | https://www.epa.gov/chemicals-under-tsca | |
Description | EPA Chemicals under the Toxic Substances Control Act (TSCA) collection contains information on chemicals and their regulations under TSCA, including non-confidential content from the TSCA Chemical Substance Inventory and Chemical Data Reporting. | |
Record name | Methane--titanium (1/1) | |
Source | EPA DSSTox | |
URL | https://comptox.epa.gov/dashboard/DTXSID60925760 | |
Description | DSSTox provides a high quality public chemistry resource for supporting improved predictive toxicology. | |
Record name | TITANIUM CARBIDE | |
Source | FDA Global Substance Registration System (GSRS) | |
URL | https://gsrs.ncats.nih.gov/ginas/app/beta/substances/7SHTGW5HBI | |
Description | The FDA Global Substance Registration System (GSRS) enables the efficient and accurate exchange of information on what substances are in regulated products. Instead of relying on names, which vary across regulatory domains, countries, and regions, the GSRS knowledge base makes it possible for substances to be defined by standardized, scientific descriptions. | |
Explanation | Unless otherwise noted, the contents of the FDA website (www.fda.gov), both text and graphics, are not copyrighted. They are in the public domain and may be republished, reprinted and otherwise used freely by anyone without the need to obtain permission from FDA. Credit to the U.S. Food and Drug Administration as the source is appreciated but not required. | |
Retrosynthesis Analysis
AI-Powered Synthesis Planning: Our tool employs the Template_relevance Pistachio, Template_relevance Bkms_metabolic, Template_relevance Pistachio_ringbreaker, Template_relevance Reaxys, Template_relevance Reaxys_biocatalysis model, leveraging a vast database of chemical reactions to predict feasible synthetic routes.
One-Step Synthesis Focus: Specifically designed for one-step synthesis, it provides concise and direct routes for your target compounds, streamlining the synthesis process.
Accurate Predictions: Utilizing the extensive PISTACHIO, BKMS_METABOLIC, PISTACHIO_RINGBREAKER, REAXYS, REAXYS_BIOCATALYSIS database, our tool offers high-accuracy predictions, reflecting the latest in chemical research and data.
Strategy Settings
Precursor scoring | Relevance Heuristic |
---|---|
Min. plausibility | 0.01 |
Model | Template_relevance |
Template Set | Pistachio/Bkms_metabolic/Pistachio_ringbreaker/Reaxys/Reaxys_biocatalysis |
Top-N result to add to graph | 6 |
Feasible Synthetic Routes
Disclaimer and Information on In-Vitro Research Products
Please be aware that all articles and product information presented on BenchChem are intended solely for informational purposes. The products available for purchase on BenchChem are specifically designed for in-vitro studies, which are conducted outside of living organisms. In-vitro studies, derived from the Latin term "in glass," involve experiments performed in controlled laboratory settings using cells or tissues. It is important to note that these products are not categorized as medicines or drugs, and they have not received approval from the FDA for the prevention, treatment, or cure of any medical condition, ailment, or disease. We must emphasize that any form of bodily introduction of these products into humans or animals is strictly prohibited by law. It is essential to adhere to these guidelines to ensure compliance with legal and ethical standards in research and experimentation.