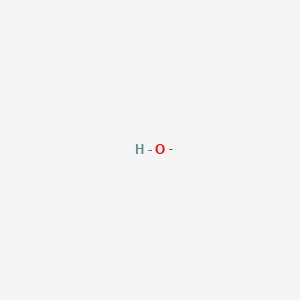
Hydroxide
- Click on QUICK INQUIRY to receive a quote from our team of experts.
- With the quality product at a COMPETITIVE price, you can focus more on your research.
Overview
Description
Hydroxide, also known as the hydroxyl ion, is a negatively charged molecule consisting of one oxygen and one hydrogen atom. It is a vital component in many chemical reactions, both inorganic and organic. Hydroxide is commonly found in solutions of bases, such as sodium hydroxide and potassium hydroxide, and is often used in laboratory experiments and scientific research.
Scientific Research Applications
Magnesium Hydroxide and Oxide
Magnesium hydroxide (Mg(OH)2) and magnesium oxide (MgO) demonstrate unique properties, making them highly applicable in various scientific fields. Their synthesis methods include precipitation, solvothermal and hydrothermal processes, sonochemical technique, sol-gel, electrochemical and microwave methods, and more. Mg(OH)2 is particularly noted for its use as an antibacterial agent, pollutant neutralizer in freshwater and wastewater, membrane component, and prominently as a flame retardant. On the other hand, MgO is characterized as an antibacterial agent, fire-resistant, and insulating material. Its uses as an adsorbent, active catalyst, and catalyst support are specially emphasized. Continuous research and modification of these compounds open new directions for their applications in advanced powder technology (Pilarska, Klapiszewski & Jesionowski, 2017).
Sodium Hydroxide in Alkaline Tank Waste
Research focused on liquid-liquid extraction chemistry relevant to the separation of major sodium salts from alkaline tank waste, emphasizing sodium hydroxide due to its value for recycling. This research is vital for evaluating the feasibility of liquid-liquid extraction chemistry for reducing the volume of tank waste. Understanding the extractive chemistry of sodium hydroxide and other sodium salts is pivotal, and the study aimed to identify selectivity, efficiency, speciation, and structure of potential extractants (Moyer, Marchand, Bonnesen, Bryan & Haverlock, 2002).
Layered Double Hydroxide Nanoparticles
Layered double hydroxide (LDH) nanomaterials are extensively studied due to their potential applications in drug and gene delivery. The emerging field of nanotoxicology necessitates the development of sensitive methodologies to track in vivo delivery kinetics, persistence, and bioaccumulation of LDH carriers. Radiolabeling through dual isomorphous substitution offers a method to track and follow the structural dissolution of these nanomaterials, providing insights into their behavior in biological systems (Musumeci, Schiller, Xu, Minchin, Martin & Smith, 2010).
Metal Hydroxides in High-Temperature Environments
Metal hydroxides, formed by the reaction of metal oxide with water vapor, are crucial in various high-temperature corrosion processes. Studying the thermodynamics of metal hydroxides through experimental methods such as gas leak Knudsen cell mass spectrometry and transpiration studies sheds light on their stability and behavior in high-temperature environments (Jacobson, Myers, Opila & Copland, 2005).
Nickel Hydroxides in Batteries and Engineering
Nickel hydroxide is an important material in engineering and physics, notably used in batteries. Research covers the structures, synthesis methods, and properties of nickel hydroxides, including their magnetic, vibrational, optical, electrical, and mechanical properties. These insights are crucial for their applications in engineering and highlight the potentially useful properties of these materials (Hall, Lockwood, Bock & Macdougall, 2015).
properties
CAS RN |
14280-30-9 |
---|---|
Product Name |
Hydroxide |
Molecular Formula |
OH(−) HO- |
Molecular Weight |
17.007 g/mol |
IUPAC Name |
hydroxide |
InChI |
InChI=1S/H2O/h1H2/p-1 |
InChI Key |
XLYOFNOQVPJJNP-UHFFFAOYSA-M |
SMILES |
[OH-] |
Canonical SMILES |
[OH-] |
Other CAS RN |
14337-01-0 14280-30-9 |
physical_description |
Solid |
synonyms |
hydroxide hydroxide ion hydroxyl ion OH- |
Origin of Product |
United States |
Retrosynthesis Analysis
AI-Powered Synthesis Planning: Our tool employs the Template_relevance Pistachio, Template_relevance Bkms_metabolic, Template_relevance Pistachio_ringbreaker, Template_relevance Reaxys, Template_relevance Reaxys_biocatalysis model, leveraging a vast database of chemical reactions to predict feasible synthetic routes.
One-Step Synthesis Focus: Specifically designed for one-step synthesis, it provides concise and direct routes for your target compounds, streamlining the synthesis process.
Accurate Predictions: Utilizing the extensive PISTACHIO, BKMS_METABOLIC, PISTACHIO_RINGBREAKER, REAXYS, REAXYS_BIOCATALYSIS database, our tool offers high-accuracy predictions, reflecting the latest in chemical research and data.
Strategy Settings
Precursor scoring | Relevance Heuristic |
---|---|
Min. plausibility | 0.01 |
Model | Template_relevance |
Template Set | Pistachio/Bkms_metabolic/Pistachio_ringbreaker/Reaxys/Reaxys_biocatalysis |
Top-N result to add to graph | 6 |
Feasible Synthetic Routes
Disclaimer and Information on In-Vitro Research Products
Please be aware that all articles and product information presented on BenchChem are intended solely for informational purposes. The products available for purchase on BenchChem are specifically designed for in-vitro studies, which are conducted outside of living organisms. In-vitro studies, derived from the Latin term "in glass," involve experiments performed in controlled laboratory settings using cells or tissues. It is important to note that these products are not categorized as medicines or drugs, and they have not received approval from the FDA for the prevention, treatment, or cure of any medical condition, ailment, or disease. We must emphasize that any form of bodily introduction of these products into humans or animals is strictly prohibited by law. It is essential to adhere to these guidelines to ensure compliance with legal and ethical standards in research and experimentation.