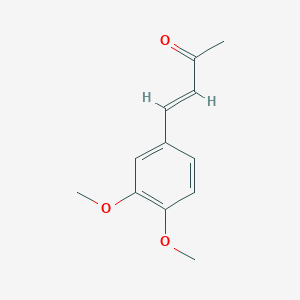
4-(3,4-Dimethoxyphenyl)-3-buten-2-one
Overview
Description
4-(3,4-Dimethoxyphenyl)-3-buten-2-one is a chalcone derivative characterized by a propenone backbone substituted with a 3,4-dimethoxyphenyl group. This compound belongs to a broader class of α,β-unsaturated ketones, which are widely studied for their diverse biological activities, including anti-inflammatory, antioxidant, and anticancer properties .
Preparation Methods
Aldol Condensation Methods
Aldol condensation between 3,4-dimethoxybenzaldehyde and acetone is the most widely employed strategy for synthesizing 4-(3,4-Dimethoxyphenyl)-3-buten-2-one. This section delineates traditional and modern variations of this approach.
Traditional Base-Catalyzed Aldol Condensation
The reaction involves deprotonating acetone to form an enolate ion, which nucleophilically attacks the electrophilic carbonyl carbon of 3,4-dimethoxybenzaldehyde. Subsequent dehydration yields the α,β-unsaturated ketone.
Procedure :
-
Catalyst : Aqueous sodium hydroxide (NaOH) or potassium hydroxide (KOH).
-
Solvent System : Ethanol-water mixtures (typically 1:1 v/v) to balance solubility and reactivity .
-
Molar Ratio : 1:1 aldehyde-to-acetone ratio to favor mono-condensation .
-
Conditions : Room temperature (RT) or mild heating (40–60°C) for 6–24 hours .
Optimization Insights :
-
Excess benzaldehyde derivatives risk di-condensed byproducts (e.g., dibenzalacetone analogs) .
-
Yields of 65–75% are typical, with purification via recrystallization from ethanol .
Microwave-Assisted Aldol Condensation
Microwave irradiation enhances reaction kinetics and selectivity by enabling rapid, uniform heating.
Procedure :
-
Catalyst : Mg–Al hydrotalcite (HT) or its calcined mixed metal oxide (MMO) .
-
Solvent : Anhydrous ethanol or toluene.
-
Conditions : Microwave reactor at 100–120°C for 10–30 minutes under 15–20 bar pressure .
Advantages :
Catalytic Methods Using Solid Catalysts
Heterogeneous catalysts offer recyclability and simplified purification. Two prominent systems are explored here.
Mesoporous Aluminosilicate Catalysts
Adapted from styrene-acetic anhydride condensation , mesoporous aluminosilicates (SiO₂/Al₂O₃ = 80) facilitate acylation reactions.
Procedure :
-
Substrate : 3,4-Dimethoxybenzaldehyde and acetic anhydride.
Outcomes :
-
Converts aldehydes to α,β-unsaturated ketones via Friedel-Crafts-type mechanisms.
-
Limited yield data for the target compound, but analogous reactions achieve ~70% efficiency .
Hydrotalcite-Based Catalysts
Mg–Al hydrotalcites, either as-synthesized or calcined, exhibit high basicity and surface area for aldol condensation .
Procedure :
-
Catalyst Activation : Calcination at 450°C transforms HT to MMO, which is rehydrated for enhanced activity .
-
Solvent-Free Conditions : Direct mixing of aldehyde, acetone, and catalyst under microwave irradiation .
Performance :
-
Conversion : >90% for furfural-acetone systems, suggesting applicability to 3,4-dimethoxybenzaldehyde .
-
Reusability : Catalysts retain >80% activity after three cycles .
Optimization of Reaction Conditions
Critical parameters influencing yield and selectivity include:
Table 1. Comparative Analysis of Preparation Methods
Method | Catalyst | Temperature (°C) | Time | Yield (%) | Selectivity |
---|---|---|---|---|---|
Traditional Aldol | NaOH | 25–60 | 6–24 h | 65–75 | Moderate |
Microwave-Assisted | Mg-Al Hydrotalcite | 100–120 | 10–30 min | 80–85 | High |
Mesoporous Catalysis | Aluminosilicate | 120–130 | 3–5 h | ~70* | Moderate |
*Estimated from analogous reactions .
Key Findings :
-
Molar Ratio : Stoichiometric aldehyde:acetone (1:1) minimizes di-condensation .
-
Catalyst Loading : 10–20 wt% optimizes cost and efficiency .
-
Solvent Choice : Ethanol enhances solubility of polar intermediates .
Mechanistic and Kinetic Considerations
Reaction Pathway
Aldol condensation proceeds via:
-
Enolate Formation : Base deprotonates acetone, generating a nucleophilic enolate .
-
Nucleophilic Attack : Enolate attacks the aldehyde carbonyl, forming a β-hydroxy ketone intermediate .
-
Dehydration : Base-catalyzed elimination of water produces the conjugated enone .
Stereochemical Outcome :
Kinetic Studies
Chemical Reactions Analysis
Types of Reactions: 4-(3,4-Dimethoxyphenyl)-3-buten-2-one can undergo various chemical reactions, including:
Oxidation: The compound can be oxidized to form corresponding carboxylic acids or ketones.
Reduction: Reduction reactions can convert the butenone group to an alcohol.
Substitution: Electrophilic aromatic substitution reactions can occur on the dimethoxyphenyl ring.
Common Reagents and Conditions:
Oxidation: Common oxidizing agents include potassium permanganate and chromium trioxide.
Reduction: Sodium borohydride or lithium aluminum hydride are typical reducing agents.
Substitution: Halogens or nitrating agents can be used for substitution reactions on the aromatic ring.
Major Products:
Oxidation: Carboxylic acids or ketones.
Reduction: Alcohols.
Substitution: Halogenated or nitrated derivatives of the original compound.
Scientific Research Applications
Pharmaceutical Applications
4-(3,4-Dimethoxyphenyl)-3-buten-2-one has been investigated for its potential therapeutic properties:
- Anti-inflammatory Activity : Studies indicate that derivatives of this compound exhibit significant anti-inflammatory effects, making them candidates for developing new anti-inflammatory drugs .
- Antioxidant Properties : The compound has shown promise as an antioxidant, which can help in preventing oxidative stress-related diseases .
- Anticancer Activity : Research highlights its potential in cancer therapy. For instance, chalcone derivatives have been noted for their ability to inhibit cancer cell proliferation .
Synthesis and Organic Chemistry
The compound serves as an important intermediate in organic synthesis:
- Synthesis of Other Compounds : It is utilized in the synthesis of various bioactive molecules and natural products, including flavonoids and other polyphenolic compounds .
- Chalcone Synthesis : As a chalcone derivative, it plays a crucial role in the synthesis of more complex aromatic compounds through methods like Claisen-Schmidt condensation .
Case Study 1: Anticancer Activity
A study published in the European Journal of Medicinal Chemistry demonstrated that derivatives of this compound exhibited potent anticancer activity against various human cancer cell lines. The mechanism was attributed to the induction of apoptosis and cell cycle arrest .
Case Study 2: Antioxidant Effects
Research conducted by Kuo et al. (2005) evaluated the antioxidant capacity of this compound and its derivatives. The findings suggested that these compounds could scavenge free radicals effectively, thus providing protective effects against oxidative damage .
Table 1: Biological Activities of this compound Derivatives
Table 2: Synthesis Pathways Involving this compound
Mechanism of Action
The mechanism of action of 4-(3,4-Dimethoxyphenyl)-3-buten-2-one involves its interaction with specific molecular targets. For instance, it may inhibit certain enzymes by binding to their active sites, thereby blocking their activity. The pathways involved can include inhibition of oxidative stress pathways or modulation of signaling pathways related to inflammation and cell proliferation .
Comparison with Similar Compounds
Comparison with Structurally Similar Compounds
Structural Analogs and Substitution Effects
Hydroxyphenyl vs. Methoxyphenyl Derivatives
- 4-(3,4-Dihydroxyphenyl)-3-buten-2-one: Isolated from Phellinus baumii and Phellinus linteus, this compound differs by replacing methoxy groups with hydroxyls. It exhibited anti-inflammatory activity by inhibiting NO production in LPS-stimulated RAW264.7 macrophages . Key Contrast: The methoxy groups in 4-(3,4-dimethoxyphenyl)-3-buten-2-one likely improve metabolic stability and membrane penetration compared to hydroxylated analogs.
- 4-(4-Hydroxyphenyl)-3-buten-2-one: Found in Phellinus species, this simpler analog lacks the 3-methoxy substitution. It showed moderate NO inhibition (IC₅₀ ~15 µM), suggesting that additional methoxy groups may amplify anti-inflammatory effects .
Halogenated and Functionalized Derivatives
- However, this may also elevate toxicity, as seen in halogenated chalcone derivatives .
- α-Fluorinated and α-Cyano Derivatives: Fluorine or cyano substitutions at the α-position (e.g., α-F-TMC and α-CN-TMC) significantly alter electronic properties and steric effects, modulating interactions with enzymes like COX-2 or kinases .
Anti-Inflammatory Activity
- COX-2 Inhibition :
- NO Production Inhibition: Lignans and diterpenes with 3,4-dimethoxyphenyl groups (e.g., vitekwangin A) from Vitex species inhibited NO production in RAW264.7 cells, with IC₅₀ values ranging from 10–50 µM .
Neurotrophic and Anticancer Effects
- Anticancer Activity :
Physicochemical and Pharmacokinetic Properties
*Predicted using QikProp (Schrödinger).
Biological Activity
4-(3,4-Dimethoxyphenyl)-3-buten-2-one, also known as DMPB, is an organic compound characterized by a butenone group attached to a dimethoxyphenyl ring. Its unique structure imparts significant biological activities, making it a subject of interest in various fields including medicinal chemistry, pharmacology, and biochemistry.
- Molecular Formula : C₁₂H₁₄O₃
- CAS Number : 60234-90-4
This compound is notable for its potential applications in organic synthesis and its diverse biological effects.
The biological activity of this compound is primarily linked to its ability to interact with specific molecular targets. Research indicates that it enhances melanogenesis by increasing the expression of tyrosinase, an enzyme crucial for melanin production. This action is mediated through the activation of signaling pathways involving upstream stimulating factor-1 (USF1) and extracellular signal-regulated kinase (ERK) .
1. Melanogenesis Enhancement
A significant study demonstrated that DMPB promotes melanin synthesis in both B16F10 melanoma cells and human primary melanocytes. The compound was shown to activate ERK and p38 pathways while enhancing tyrosinase levels. In vivo studies indicated that DMPB induced hyperpigmentation in guinea pigs, suggesting its potential therapeutic use against hypopigmentation disorders .
2. Antioxidant Properties
The antioxidant activity of DMPB has been evaluated using various assays such as DPPH and FRAP methods. Its structural features contribute to its ability to scavenge free radicals effectively, highlighting its potential protective role against oxidative stress .
3. Antifungal Activity
DMPB has exhibited antifungal properties, particularly against wood-decay fungi. This activity suggests its potential application in preserving wood products and as a natural fungicide .
Case Studies
Comparative Analysis with Similar Compounds
To better understand the unique biological activity of DMPB, it is useful to compare it with structurally similar compounds.
Compound | Structure | Biological Activity |
---|---|---|
4-(3,4-Dimethoxyphenyl)-3-buten-2-ol | Hydroxyl group instead of ketone | Similar melanogenesis enhancement but lower potency . |
3,4-Dimethoxyphenethylamine | Analog with different functional groups | Neuroactive properties but lacks significant melanogenic activity . |
Q & A
Basic Research Questions
Q. What synthetic methodologies are recommended for 4-(3,4-Dimethoxyphenyl)-3-buten-2-one, and how can reaction efficiency be optimized?
- Methodological Answer : Claisen-Schmidt condensation is a common approach for α,β-unsaturated ketones. For this compound, optimize solvent polarity (e.g., ethanol or THF), base selection (e.g., NaOH or KOH), and stoichiometric ratios of 3,4-dimethoxybenzaldehyde and acetone derivatives. Monitor reaction progress via TLC and purify using column chromatography with silica gel (60–120 mesh). Post-synthetic validation via H NMR (aromatic protons at δ 6.7–7.2 ppm) and GC-MS (molecular ion peak at m/z 220) is critical .
Q. Which spectroscopic techniques are most effective for structural characterization of this compound?
- Methodological Answer :
- H/C NMR : Confirm aromatic substitution patterns (e.g., methoxy groups at δ 3.8–3.9 ppm) and α,β-unsaturated carbonyl resonance (δ 7.5–8.0 ppm for vinyl protons).
- IR Spectroscopy : Identify carbonyl stretching (~1680 cm) and conjugated C=C bonds (~1600 cm).
- GC-MS : Use a non-polar column (e.g., HP-5MS) with temperature programming (60°C to 280°C at 5–10°C/min) for retention time alignment with NIST reference data .
Q. What safety protocols are essential for handling this compound in laboratory settings?
- Methodological Answer : Use PPE (nitrile gloves, lab coat), fume hoods for ventilation, and P95 respirators during prolonged exposure. Store in amber glass bottles at 4°C under inert atmosphere (N) to prevent oxidation. Emergency procedures: For skin contact, wash with 10% sodium bicarbonate; for eye exposure, irrigate with saline for 15 minutes .
Advanced Research Questions
Q. How can researchers resolve contradictions in reported bioactivity data across studies?
- Methodological Answer :
- Standardized Assays : Use cell lines with consistent passage numbers and validate purity (>98% via HPLC).
- Control Degradation : Monitor sample stability under assay conditions (e.g., 37°C, pH 7.4) using LC-MS to detect degradation products.
- Statistical Validation : Apply ANOVA or Tukey’s HSD test to compare inter-study variability, accounting for batch effects .
Q. What experimental designs mitigate oxidative degradation during long-term stability studies?
- Methodological Answer :
- Accelerated Degradation Studies : Expose samples to 40°C/75% RH for 6 months, analyzing degradation kinetics via Arrhenius plots.
- Stabilization Strategies : Add antioxidants (e.g., BHT at 0.01% w/v) or use amber vials with oxygen scavengers.
- Analytical Monitoring : Quantify degradation products (e.g., quinone derivatives) using UPLC-PDA at 254 nm .
Q. How can computational modeling predict interaction mechanisms with biological targets?
- Methodological Answer :
- Molecular Docking : Use AutoDock Vina to simulate binding to cyclooxygenase-2 (COX-2) or NF-κB, focusing on methoxy group interactions with hydrophobic pockets.
- MD Simulations : Run 100-ns simulations in GROMACS to assess stability of ligand-protein complexes (RMSD < 2 Å). Validate predictions with SPR binding assays (KD < 10 μM threshold) .
Q. Data Analysis & Validation
Q. What statistical approaches are recommended for validating reproducibility in synthetic yields?
- Methodological Answer : Perform triplicate syntheses under identical conditions. Calculate % yield mean ± SD and apply Grubbs’ test to exclude outliers. Use a t-test (p < 0.05) to compare yields across catalyst systems (e.g., homogeneous vs. heterogeneous) .
Q. How can researchers optimize HPLC methods for quantifying trace impurities?
- Methodological Answer :
- Column : C18 (5 μm, 250 × 4.6 mm) with mobile phase gradient (acetonitrile:water 40:60 to 80:20 in 20 min).
- Detection : UV at 280 nm for carbonyl chromophores. Validate LOD/LOQ (e.g., 0.1 μg/mL and 0.3 μg/mL via signal-to-noise ratio).
- Forced Degradation : Expose to UV light (254 nm, 48 hr) and acidic/alkaline hydrolysis to identify impurity profiles .
Q. Experimental Design Limitations
Q. What are the limitations of using simplified models (e.g., single-pollutant systems) to predict environmental behavior?
- Methodological Answer : Simplified models ignore matrix effects (e.g., organic matter interactions). Improve generalizability by spiking real wastewater samples and analyzing via hyphenated techniques (HSI-TOF-MS) to capture co-pollutant interactions. Include kinetic studies to assess photodegradation half-lives in natural sunlight .
Properties
IUPAC Name |
(E)-4-(3,4-dimethoxyphenyl)but-3-en-2-one | |
---|---|---|
Source | PubChem | |
URL | https://pubchem.ncbi.nlm.nih.gov | |
Description | Data deposited in or computed by PubChem | |
InChI |
InChI=1S/C12H14O3/c1-9(13)4-5-10-6-7-11(14-2)12(8-10)15-3/h4-8H,1-3H3/b5-4+ | |
Source | PubChem | |
URL | https://pubchem.ncbi.nlm.nih.gov | |
Description | Data deposited in or computed by PubChem | |
InChI Key |
XUYBNDHXZMIALN-SNAWJCMRSA-N | |
Source | PubChem | |
URL | https://pubchem.ncbi.nlm.nih.gov | |
Description | Data deposited in or computed by PubChem | |
Canonical SMILES |
CC(=O)C=CC1=CC(=C(C=C1)OC)OC | |
Source | PubChem | |
URL | https://pubchem.ncbi.nlm.nih.gov | |
Description | Data deposited in or computed by PubChem | |
Isomeric SMILES |
CC(=O)/C=C/C1=CC(=C(C=C1)OC)OC | |
Source | PubChem | |
URL | https://pubchem.ncbi.nlm.nih.gov | |
Description | Data deposited in or computed by PubChem | |
Molecular Formula |
C12H14O3 | |
Source | PubChem | |
URL | https://pubchem.ncbi.nlm.nih.gov | |
Description | Data deposited in or computed by PubChem | |
Molecular Weight |
206.24 g/mol | |
Source | PubChem | |
URL | https://pubchem.ncbi.nlm.nih.gov | |
Description | Data deposited in or computed by PubChem | |
CAS No. |
60234-90-4, 15001-27-1 | |
Record name | Veratralacetone | |
Source | ChemIDplus | |
URL | https://pubchem.ncbi.nlm.nih.gov/substance/?source=chemidplus&sourceid=0060234904 | |
Description | ChemIDplus is a free, web search system that provides access to the structure and nomenclature authority files used for the identification of chemical substances cited in National Library of Medicine (NLM) databases, including the TOXNET system. | |
Record name | 15001-27-1 | |
Source | DTP/NCI | |
URL | https://dtp.cancer.gov/dtpstandard/servlet/dwindex?searchtype=NSC&outputformat=html&searchlist=217305 | |
Description | The NCI Development Therapeutics Program (DTP) provides services and resources to the academic and private-sector research communities worldwide to facilitate the discovery and development of new cancer therapeutic agents. | |
Explanation | Unless otherwise indicated, all text within NCI products is free of copyright and may be reused without our permission. Credit the National Cancer Institute as the source. | |
Record name | 4-(3,4-dimethoxyphenyl)-3-buten-2-one | |
Source | European Chemicals Agency (ECHA) | |
URL | https://echa.europa.eu/substance-information/-/substanceinfo/100.035.526 | |
Description | The European Chemicals Agency (ECHA) is an agency of the European Union which is the driving force among regulatory authorities in implementing the EU's groundbreaking chemicals legislation for the benefit of human health and the environment as well as for innovation and competitiveness. | |
Explanation | Use of the information, documents and data from the ECHA website is subject to the terms and conditions of this Legal Notice, and subject to other binding limitations provided for under applicable law, the information, documents and data made available on the ECHA website may be reproduced, distributed and/or used, totally or in part, for non-commercial purposes provided that ECHA is acknowledged as the source: "Source: European Chemicals Agency, http://echa.europa.eu/". Such acknowledgement must be included in each copy of the material. ECHA permits and encourages organisations and individuals to create links to the ECHA website under the following cumulative conditions: Links can only be made to webpages that provide a link to the Legal Notice page. | |
Record name | VERATRALACETONE | |
Source | FDA Global Substance Registration System (GSRS) | |
URL | https://gsrs.ncats.nih.gov/ginas/app/beta/substances/DBT8CR6TVM | |
Description | The FDA Global Substance Registration System (GSRS) enables the efficient and accurate exchange of information on what substances are in regulated products. Instead of relying on names, which vary across regulatory domains, countries, and regions, the GSRS knowledge base makes it possible for substances to be defined by standardized, scientific descriptions. | |
Explanation | Unless otherwise noted, the contents of the FDA website (www.fda.gov), both text and graphics, are not copyrighted. They are in the public domain and may be republished, reprinted and otherwise used freely by anyone without the need to obtain permission from FDA. Credit to the U.S. Food and Drug Administration as the source is appreciated but not required. | |
Retrosynthesis Analysis
AI-Powered Synthesis Planning: Our tool employs the Template_relevance Pistachio, Template_relevance Bkms_metabolic, Template_relevance Pistachio_ringbreaker, Template_relevance Reaxys, Template_relevance Reaxys_biocatalysis model, leveraging a vast database of chemical reactions to predict feasible synthetic routes.
One-Step Synthesis Focus: Specifically designed for one-step synthesis, it provides concise and direct routes for your target compounds, streamlining the synthesis process.
Accurate Predictions: Utilizing the extensive PISTACHIO, BKMS_METABOLIC, PISTACHIO_RINGBREAKER, REAXYS, REAXYS_BIOCATALYSIS database, our tool offers high-accuracy predictions, reflecting the latest in chemical research and data.
Strategy Settings
Precursor scoring | Relevance Heuristic |
---|---|
Min. plausibility | 0.01 |
Model | Template_relevance |
Template Set | Pistachio/Bkms_metabolic/Pistachio_ringbreaker/Reaxys/Reaxys_biocatalysis |
Top-N result to add to graph | 6 |
Feasible Synthetic Routes
Disclaimer and Information on In-Vitro Research Products
Please be aware that all articles and product information presented on BenchChem are intended solely for informational purposes. The products available for purchase on BenchChem are specifically designed for in-vitro studies, which are conducted outside of living organisms. In-vitro studies, derived from the Latin term "in glass," involve experiments performed in controlled laboratory settings using cells or tissues. It is important to note that these products are not categorized as medicines or drugs, and they have not received approval from the FDA for the prevention, treatment, or cure of any medical condition, ailment, or disease. We must emphasize that any form of bodily introduction of these products into humans or animals is strictly prohibited by law. It is essential to adhere to these guidelines to ensure compliance with legal and ethical standards in research and experimentation.