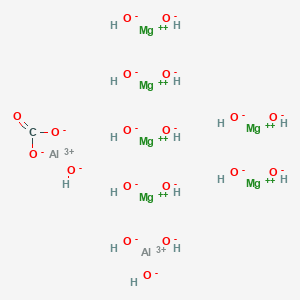
Aluminum magnesium hydroxide carbonate
Overview
Description
Synthesis Analysis
AMHC can be synthesized using several methods, with a focus on producing pure crystals and optimizing particle size. A notable approach involves using aluminum sulfate as an aluminum source in a solid-state reaction to successfully prepare magnesium aluminum carbonate hydroxide (Wu Zhi-hong & Liao Sen, 2004). Another method synthesizes layered magnesium-aluminum hydroxides on the surface of γ-Al2O3, highlighting the versatility in synthetic approaches to create structures with varying properties (O. Belskaya et al., 2019).
Molecular Structure Analysis
AMHC's structure is characterized by its layered double hydroxide nature, allowing for the intercalation of various anions, including carbonate. This structure is versatile and can be tailored through synthetic methods to achieve specific molecular arrangements and properties. The interlayer spacing and the presence of carbonate ions are crucial for its structural characteristics and reactivity (V. Matveev et al., 2021).
Chemical Reactions and Properties
AMHC participates in various chemical reactions due to its reactive sites and the ability to exchange interlayer anions. Its synthesis often involves reactions between magnesium and aluminum hydroxides with carbonate ions under specific conditions to form the layered structure. These reactions are influenced by factors such as temperature, pH, and the presence of other ions, which can affect the crystallinity and purity of the final product (V. Khusnutdinov & V. Isupov, 2008).
Physical Properties Analysis
The physical properties of AMHC, such as particle size, morphology, and crystallinity, are critical for its applications and can be controlled through synthesis. Techniques like solid-state reaction and hydrothermal methods can optimize these properties, leading to the production of nano-crystals with specific surface characteristics (Zhi-hong Wu, 2004).
Chemical Properties Analysis
AMHC's chemical properties are significantly influenced by its layered structure and the presence of carbonate ions. These aspects contribute to its reactivity, ion-exchange capacity, and ability to interact with various chemical species. Its utility in catalysis, adsorption, and as a precursor for other materials stems from these chemical characteristics, which can be tailored through different synthesis approaches (He Yan-ting, 2005).
Scientific Research Applications
Corrosion Resistance : Magnesium-aluminum layered double hydroxide films can be used to protect magnesium alloys from corrosion. This is achieved through a hydrothermal crystallization method, resulting in a uniform and compact LDH film with strong adhesion to the substrate, enhancing its practical application potential (Wang et al., 2010).
Catalysis : LDHs containing interlayer carbonate counterions have been studied as precursors for supports in platinum catalysts. The nature of the interlayer anion significantly affects the formation of the oxide support and its texture characteristics, influencing its catalytic properties (Belskaya et al., 2011).
Biomedical Applications : Magnesium hydroxide, a component of hydrotalcite, has been used as a phosphate binder in patients on hemodialysis. Its effectiveness in reducing the required dose of aluminum hydroxide and its impact on parathyroid hormone concentration suggest potential in medical applications (Oe et al., 1987).
Environmental Remediation : Magnesium-aluminum layered double hydroxides intercalated with nitrogen-containing anions show enhanced adsorption activities for CO2, particularly in natural gas streams. This application is significant for CO2 capture and environmental remediation (Faramawy et al., 2018).
Substitution and Modification Studies : Research has been conducted on the substitution of aluminum by bismuth in magnesium-aluminum-bismuth-layered double hydroxides, showing that a maximum rate of substitution of about 20 mol.% can be achieved. This has implications for the modification of LDH properties for specific applications (Sokol et al., 2017).
Material Synthesis and Transformation : Studies on the transformation mechanism of magnesium and aluminum precursor solutions into crystallites of layered double hydroxide provide insight into the synthesis methodologies and potential for tailoring LDH properties for various industrial applications (Yang et al., 2012).
Ion-Exchange and Adsorption Properties : The anion-exchange ability of LDHs has been explored for applications in catalysis, drug delivery, and environmental remediation. Understanding the specific cation arrangements in LDHs enhances the functional optimization of these materials (Sideris et al., 2008).
Mechanism of Action
Target of Action
The primary targets of Aluminum Magnesium Hydroxide Carbonate are the excess acids in the stomach. These compounds are used as antacids, which are substances that neutralize stomach acidity and are used to relieve symptoms such as heartburn and indigestion .
Mode of Action
This compound acts by neutralizing gastric acid . Aluminum hydroxide is a slow-acting antacid that binds phosphate in the gastrointestinal tract to form insoluble complexes, reducing phosphate absorption . On the other hand, magnesium hydroxide is a fast-acting antacid which counteracts the constipating effect of aluminum hydroxide . These compounds react with gastric hydrochloric acid (HCl) to form water and a salt, thereby lowering the acidity in the stomach .
Biochemical Pathways
The action of this compound affects the gastric acid secretion pathway . By neutralizing gastric acid, these compounds increase the pH of the stomach, which may inhibit the action of pepsin, an enzyme involved in protein digestion . An increase in bicarbonate ions and prostaglandins may also confer cytoprotective effects .
Pharmacokinetics
Aluminum hydroxide is slowly solubilized in the stomach and reacts with hydrochloric acid to form aluminum chloride and water . It also inhibits the action of pepsin by increasing the pH and via adsorption . The pharmacokinetics of these compounds are influenced by their rate of dissolution and their interaction with other substances in the gastrointestinal tract .
Result of Action
The result of the action of this compound is the relief of symptoms associated with excess stomach acid, such as heartburn, acid indigestion, and sour stomach . By neutralizing stomach acid, these compounds can help to prevent damage to the stomach lining caused by acid.
Action Environment
The action of this compound can be influenced by various environmental factors. For instance, the presence of CO2 plays a key role in the corrosion of magnesium, which can affect the stability of these compounds . Additionally, the presence of other ions in the stomach, such as phosphate, can influence the action of these compounds .
Safety and Hazards
When handling Aluminum magnesium hydroxide carbonate, it is advised to avoid dust formation, breathing mist, gas or vapours, and contacting with skin and eye. Use personal protective equipment, wear chemical impermeable gloves, ensure adequate ventilation, remove all sources of ignition, evacuate personnel to safe areas, and keep people away from and upwind of spill/leak .
Future Directions
While Aluminum magnesium hydroxide carbonate is currently used as an antacid and in food-contact polymers , future research may explore other potential applications. For instance, its use in the treatment of other medical conditions or its potential in various industrial applications could be investigated.
properties
IUPAC Name |
dialuminum;hexamagnesium;carbonate;hexadecahydroxide | |
---|---|---|
Source | PubChem | |
URL | https://pubchem.ncbi.nlm.nih.gov | |
Description | Data deposited in or computed by PubChem | |
InChI |
InChI=1S/CH2O3.2Al.6Mg.16H2O/c2-1(3)4;;;;;;;;;;;;;;;;;;;;;;;;/h(H2,2,3,4);;;;;;;;;16*1H2/q;2*+3;6*+2;;;;;;;;;;;;;;;;/p-18 | |
Source | PubChem | |
URL | https://pubchem.ncbi.nlm.nih.gov | |
Description | Data deposited in or computed by PubChem | |
InChI Key |
GDVKFRBCXAPAQJ-UHFFFAOYSA-A | |
Source | PubChem | |
URL | https://pubchem.ncbi.nlm.nih.gov | |
Description | Data deposited in or computed by PubChem | |
Canonical SMILES |
C(=O)([O-])[O-].[OH-].[OH-].[OH-].[OH-].[OH-].[OH-].[OH-].[OH-].[OH-].[OH-].[OH-].[OH-].[OH-].[OH-].[OH-].[OH-].[Mg+2].[Mg+2].[Mg+2].[Mg+2].[Mg+2].[Mg+2].[Al+3].[Al+3] | |
Source | PubChem | |
URL | https://pubchem.ncbi.nlm.nih.gov | |
Description | Data deposited in or computed by PubChem | |
Molecular Formula |
CH16Al2Mg6O19 | |
Source | PubChem | |
URL | https://pubchem.ncbi.nlm.nih.gov | |
Description | Data deposited in or computed by PubChem | |
Molecular Weight |
531.92 g/mol | |
Source | PubChem | |
URL | https://pubchem.ncbi.nlm.nih.gov | |
Description | Data deposited in or computed by PubChem | |
Physical Description |
Dry Powder; Other Solid; Pellets or Large Crystals | |
Record name | Aluminate (Al(OH)63-), (OC-6-11)-, magnesium carbonate hydroxide (2:6:1:4) | |
Source | EPA Chemicals under the TSCA | |
URL | https://www.epa.gov/chemicals-under-tsca | |
Description | EPA Chemicals under the Toxic Substances Control Act (TSCA) collection contains information on chemicals and their regulations under TSCA, including non-confidential content from the TSCA Chemical Substance Inventory and Chemical Data Reporting. | |
CAS RN |
11097-59-9 | |
Record name | Magnesium aluminum hydroxide carbonate | |
Source | ChemIDplus | |
URL | https://pubchem.ncbi.nlm.nih.gov/substance/?source=chemidplus&sourceid=0011097599 | |
Description | ChemIDplus is a free, web search system that provides access to the structure and nomenclature authority files used for the identification of chemical substances cited in National Library of Medicine (NLM) databases, including the TOXNET system. | |
Record name | Aluminate (Al(OH)63-), (OC-6-11)-, magnesium carbonate hydroxide (2:6:1:4) | |
Source | EPA Chemicals under the TSCA | |
URL | https://www.epa.gov/chemicals-under-tsca | |
Description | EPA Chemicals under the Toxic Substances Control Act (TSCA) collection contains information on chemicals and their regulations under TSCA, including non-confidential content from the TSCA Chemical Substance Inventory and Chemical Data Reporting. | |
Record name | [carbonato(2-)]hexadecahydroxybis(aluminium)hexamagnesium | |
Source | European Chemicals Agency (ECHA) | |
URL | https://echa.europa.eu/substance-information/-/substanceinfo/100.031.187 | |
Description | The European Chemicals Agency (ECHA) is an agency of the European Union which is the driving force among regulatory authorities in implementing the EU's groundbreaking chemicals legislation for the benefit of human health and the environment as well as for innovation and competitiveness. | |
Explanation | Use of the information, documents and data from the ECHA website is subject to the terms and conditions of this Legal Notice, and subject to other binding limitations provided for under applicable law, the information, documents and data made available on the ECHA website may be reproduced, distributed and/or used, totally or in part, for non-commercial purposes provided that ECHA is acknowledged as the source: "Source: European Chemicals Agency, http://echa.europa.eu/". Such acknowledgement must be included in each copy of the material. ECHA permits and encourages organisations and individuals to create links to the ECHA website under the following cumulative conditions: Links can only be made to webpages that provide a link to the Legal Notice page. | |
Record name | ALUMINUM MAGNESIUM HYDROXIDE CARBONATE | |
Source | FDA Global Substance Registration System (GSRS) | |
URL | https://gsrs.ncats.nih.gov/ginas/app/beta/substances/RFJ7QXF345 | |
Description | The FDA Global Substance Registration System (GSRS) enables the efficient and accurate exchange of information on what substances are in regulated products. Instead of relying on names, which vary across regulatory domains, countries, and regions, the GSRS knowledge base makes it possible for substances to be defined by standardized, scientific descriptions. | |
Explanation | Unless otherwise noted, the contents of the FDA website (www.fda.gov), both text and graphics, are not copyrighted. They are in the public domain and may be republished, reprinted and otherwise used freely by anyone without the need to obtain permission from FDA. Credit to the U.S. Food and Drug Administration as the source is appreciated but not required. | |
Retrosynthesis Analysis
AI-Powered Synthesis Planning: Our tool employs the Template_relevance Pistachio, Template_relevance Bkms_metabolic, Template_relevance Pistachio_ringbreaker, Template_relevance Reaxys, Template_relevance Reaxys_biocatalysis model, leveraging a vast database of chemical reactions to predict feasible synthetic routes.
One-Step Synthesis Focus: Specifically designed for one-step synthesis, it provides concise and direct routes for your target compounds, streamlining the synthesis process.
Accurate Predictions: Utilizing the extensive PISTACHIO, BKMS_METABOLIC, PISTACHIO_RINGBREAKER, REAXYS, REAXYS_BIOCATALYSIS database, our tool offers high-accuracy predictions, reflecting the latest in chemical research and data.
Strategy Settings
Precursor scoring | Relevance Heuristic |
---|---|
Min. plausibility | 0.01 |
Model | Template_relevance |
Template Set | Pistachio/Bkms_metabolic/Pistachio_ringbreaker/Reaxys/Reaxys_biocatalysis |
Top-N result to add to graph | 6 |
Feasible Synthetic Routes
Disclaimer and Information on In-Vitro Research Products
Please be aware that all articles and product information presented on BenchChem are intended solely for informational purposes. The products available for purchase on BenchChem are specifically designed for in-vitro studies, which are conducted outside of living organisms. In-vitro studies, derived from the Latin term "in glass," involve experiments performed in controlled laboratory settings using cells or tissues. It is important to note that these products are not categorized as medicines or drugs, and they have not received approval from the FDA for the prevention, treatment, or cure of any medical condition, ailment, or disease. We must emphasize that any form of bodily introduction of these products into humans or animals is strictly prohibited by law. It is essential to adhere to these guidelines to ensure compliance with legal and ethical standards in research and experimentation.