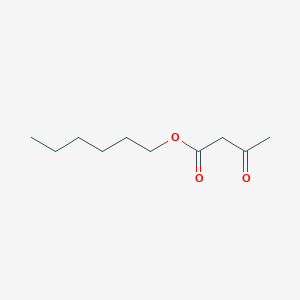
Hexyl acetoacetate
Overview
Description
Hexyl acetoacetate, also known as acetoacetic acid hexyl ester, is an organic compound with the molecular formula C10H18O3. It is a colorless to light yellow liquid with a fruity odor. This compound is commonly used in the fragrance and flavor industry due to its pleasant aroma. It is also utilized as an intermediate in organic synthesis.
Mechanism of Action
Target of Action
Hexyl acetoacetate, also known as Acetoacetic Acid Hexyl Ester , is a biochemical compound used in proteomics research
Mode of Action
Acetoacetate derivatives, such as ethyl acetoacetate, undergo a process called the Acetoacetic Ester Synthesis . This process involves the formation of an enolate ion, which can act as a nucleophile and undergo an SN2 reaction with alkyl halides, acyl (acid) chlorides, and more . After alkylation, the product can be converted to a dicarboxylic acid through saponification, and subsequently, one of the carboxylic acids can be removed through a decarboxylation step .
Biochemical Pathways
The Acetoacetic Ester Synthesis, which is likely involved in the action of this compound, is part of the broader acetyl CoA pathway . This pathway requires approximately 10 enzymes and several organic cofactors to catalyze the conversion of H2 and CO2 to formate, acetate, and pyruvate . The acetyl CoA pathway is ancient and conserved, playing a crucial role in the metabolism of many organisms .
Pharmacokinetics
As a small molecule with a molecular weight of 18625 , it can be hypothesized that it may be readily absorbed and distributed in the body. Its metabolism and excretion would likely involve enzymatic processes and renal clearance, respectively.
Result of Action
The result of the Acetoacetic Ester Synthesis, which this compound likely undergoes, is the formation of a ketone or more specifically an α-substituted acetone . This process allows the creation of complex molecules from simpler ones, which can be crucial in various biochemical processes.
Biochemical Analysis
Biochemical Properties
Hexyl Acetoacetate, as a derivative of acetoacetic acid, plays a role in biochemical reactions. It interacts with enzymes, proteins, and other biomolecules in the context of acetoacetic ester synthesis . The nature of these interactions involves the conversion of ethyl acetoacetate into a methyl ketone, a process that is facilitated by a strong base .
Cellular Effects
For instance, acetoacetate is regarded as a direct energy precursor through the TCA cycle and respiratory chain, thereby affecting cell viability .
Molecular Mechanism
The molecular mechanism of this compound involves its conversion into a methyl ketone through the Acetoacetic Ester Synthesis . This process involves the alkylation of an active methylene enolate, hydrolysis of an ester to a carboxylic acid, and decarboxylation of a β-ketoacid .
Metabolic Pathways
This compound is involved in the Acetoacetic Ester Synthesis, a metabolic pathway that involves the conversion of ethyl acetoacetate into a methyl ketone . This process involves several enzymes and results in the formation of a β-ketoacid, which is then decarboxylated .
Transport and Distribution
Given its role in the Acetoacetic Ester Synthesis, it can be inferred that it is transported and distributed in a manner that facilitates this process .
Preparation Methods
Synthetic Routes and Reaction Conditions: Hexyl acetoacetate can be synthesized through the esterification of acetoacetic acid with hexanol. The reaction typically involves the use of an acid catalyst, such as sulfuric acid, to facilitate the esterification process. The reaction is carried out under reflux conditions to ensure complete conversion of the reactants to the desired ester.
Industrial Production Methods: In an industrial setting, this compound is produced by the transesterification of ethyl acetoacetate with hexanol. This method involves the use of a catalyst, such as sodium ethoxide, to promote the transesterification reaction. The reaction is conducted at elevated temperatures to achieve high yields of the product.
Chemical Reactions Analysis
Types of Reactions: Hexyl acetoacetate undergoes various chemical reactions, including:
Oxidation: It can be oxidized to form this compound derivatives.
Reduction: Reduction reactions can convert this compound to its corresponding alcohol.
Substitution: The ester group in this compound can be substituted with other functional groups through nucleophilic substitution reactions.
Common Reagents and Conditions:
Oxidation: Common oxidizing agents include potassium permanganate and chromium trioxide.
Reduction: Reducing agents such as lithium aluminum hydride or sodium borohydride are used.
Substitution: Nucleophiles like amines or alcohols can be used under basic or acidic conditions.
Major Products Formed:
Oxidation: this compound derivatives with additional oxygen-containing functional groups.
Reduction: Hexyl alcohol.
Substitution: Various substituted esters depending on the nucleophile used.
Scientific Research Applications
Hexyl acetoacetate has several applications in scientific research:
Chemistry: It is used as an intermediate in the synthesis of various organic compounds, including pharmaceuticals and agrochemicals.
Biology: this compound is studied for its potential biological activities, such as antimicrobial and antioxidant properties.
Medicine: Research is ongoing to explore its potential use in drug development and as a bioactive compound.
Industry: It is used in the production of fragrances, flavors, and as a solvent in various industrial processes.
Comparison with Similar Compounds
Hexyl acetoacetate can be compared with other similar compounds, such as:
Ethyl acetoacetate: Both compounds are esters of acetoacetic acid, but this compound has a longer carbon chain, making it more hydrophobic.
Methyl acetoacetate: Similar to ethyl acetoacetate, but with a shorter carbon chain, resulting in different physical and chemical properties.
Butyl acetoacetate: Another ester of acetoacetic acid with a different alkyl group, leading to variations in reactivity and applications.
This compound is unique due to its specific alkyl group, which imparts distinct properties and applications compared to other acetoacetate esters.
Properties
IUPAC Name |
hexyl 3-oxobutanoate | |
---|---|---|
Source | PubChem | |
URL | https://pubchem.ncbi.nlm.nih.gov | |
Description | Data deposited in or computed by PubChem | |
InChI |
InChI=1S/C10H18O3/c1-3-4-5-6-7-13-10(12)8-9(2)11/h3-8H2,1-2H3 | |
Source | PubChem | |
URL | https://pubchem.ncbi.nlm.nih.gov | |
Description | Data deposited in or computed by PubChem | |
InChI Key |
QNZLAXONNWOLJY-UHFFFAOYSA-N | |
Source | PubChem | |
URL | https://pubchem.ncbi.nlm.nih.gov | |
Description | Data deposited in or computed by PubChem | |
Canonical SMILES |
CCCCCCOC(=O)CC(=O)C | |
Source | PubChem | |
URL | https://pubchem.ncbi.nlm.nih.gov | |
Description | Data deposited in or computed by PubChem | |
Molecular Formula |
C10H18O3 | |
Source | PubChem | |
URL | https://pubchem.ncbi.nlm.nih.gov | |
Description | Data deposited in or computed by PubChem | |
DSSTOX Substance ID |
DTXSID3065534 | |
Record name | Hexyl acetoacetate | |
Source | EPA DSSTox | |
URL | https://comptox.epa.gov/dashboard/DTXSID3065534 | |
Description | DSSTox provides a high quality public chemistry resource for supporting improved predictive toxicology. | |
Molecular Weight |
186.25 g/mol | |
Source | PubChem | |
URL | https://pubchem.ncbi.nlm.nih.gov | |
Description | Data deposited in or computed by PubChem | |
CAS No. |
13562-84-0 | |
Record name | Hexyl 3-oxobutanoate | |
Source | CAS Common Chemistry | |
URL | https://commonchemistry.cas.org/detail?cas_rn=13562-84-0 | |
Description | CAS Common Chemistry is an open community resource for accessing chemical information. Nearly 500,000 chemical substances from CAS REGISTRY cover areas of community interest, including common and frequently regulated chemicals, and those relevant to high school and undergraduate chemistry classes. This chemical information, curated by our expert scientists, is provided in alignment with our mission as a division of the American Chemical Society. | |
Explanation | The data from CAS Common Chemistry is provided under a CC-BY-NC 4.0 license, unless otherwise stated. | |
Record name | Butanoic acid, 3-oxo-, hexyl ester | |
Source | ChemIDplus | |
URL | https://pubchem.ncbi.nlm.nih.gov/substance/?source=chemidplus&sourceid=0013562840 | |
Description | ChemIDplus is a free, web search system that provides access to the structure and nomenclature authority files used for the identification of chemical substances cited in National Library of Medicine (NLM) databases, including the TOXNET system. | |
Record name | Butanoic acid, 3-oxo-, hexyl ester | |
Source | EPA Chemicals under the TSCA | |
URL | https://www.epa.gov/chemicals-under-tsca | |
Description | EPA Chemicals under the Toxic Substances Control Act (TSCA) collection contains information on chemicals and their regulations under TSCA, including non-confidential content from the TSCA Chemical Substance Inventory and Chemical Data Reporting. | |
Record name | Hexyl acetoacetate | |
Source | EPA DSSTox | |
URL | https://comptox.epa.gov/dashboard/DTXSID3065534 | |
Description | DSSTox provides a high quality public chemistry resource for supporting improved predictive toxicology. | |
Record name | Hexyl acetoacetate | |
Source | European Chemicals Agency (ECHA) | |
URL | https://echa.europa.eu/substance-information/-/substanceinfo/100.033.579 | |
Description | The European Chemicals Agency (ECHA) is an agency of the European Union which is the driving force among regulatory authorities in implementing the EU's groundbreaking chemicals legislation for the benefit of human health and the environment as well as for innovation and competitiveness. | |
Explanation | Use of the information, documents and data from the ECHA website is subject to the terms and conditions of this Legal Notice, and subject to other binding limitations provided for under applicable law, the information, documents and data made available on the ECHA website may be reproduced, distributed and/or used, totally or in part, for non-commercial purposes provided that ECHA is acknowledged as the source: "Source: European Chemicals Agency, http://echa.europa.eu/". Such acknowledgement must be included in each copy of the material. ECHA permits and encourages organisations and individuals to create links to the ECHA website under the following cumulative conditions: Links can only be made to webpages that provide a link to the Legal Notice page. | |
Retrosynthesis Analysis
AI-Powered Synthesis Planning: Our tool employs the Template_relevance Pistachio, Template_relevance Bkms_metabolic, Template_relevance Pistachio_ringbreaker, Template_relevance Reaxys, Template_relevance Reaxys_biocatalysis model, leveraging a vast database of chemical reactions to predict feasible synthetic routes.
One-Step Synthesis Focus: Specifically designed for one-step synthesis, it provides concise and direct routes for your target compounds, streamlining the synthesis process.
Accurate Predictions: Utilizing the extensive PISTACHIO, BKMS_METABOLIC, PISTACHIO_RINGBREAKER, REAXYS, REAXYS_BIOCATALYSIS database, our tool offers high-accuracy predictions, reflecting the latest in chemical research and data.
Strategy Settings
Precursor scoring | Relevance Heuristic |
---|---|
Min. plausibility | 0.01 |
Model | Template_relevance |
Template Set | Pistachio/Bkms_metabolic/Pistachio_ringbreaker/Reaxys/Reaxys_biocatalysis |
Top-N result to add to graph | 6 |
Feasible Synthetic Routes
Q1: What is the role of hexyl acetoacetate in the development of efficient organic light-emitting diodes (OLEDs)?
A1: this compound is a key component of a crosslinkable iridium(III) complex used in the emissive layer of highly efficient phosphorescent OLEDs []. Specifically, it acts as a ligand within the iridium complex, denoted as “x-emitter” in the research. This complex is designed to overcome the limitations of earlier devices by allowing for the deposition of additional layers and enhancing morphological stability through crosslinking [].
Q2: How does the crosslinking process involving this compound contribute to OLED performance?
A2: The x-emitter containing this compound can be co-polymerized with a crosslinkable matrix material, like OTPD, within the OLED's emissive layer []. This crosslinking results in an insoluble emissive layer, enabling the deposition of additional layers, such as an electron-transporting layer (ETL), on top []. The ETL improves charge balance within the device and reduces luminescence quenching at the cathode, significantly enhancing the overall efficiency of the OLED [].
Q3: Beyond OLEDs, has this compound been implicated in other biological applications?
A3: Interestingly, research suggests that this compound, among other metabolites produced by certain Trichoderma fungi species, can trigger aggregation behavior in Formosan subterranean termites (Coptotermes formosanus) []. Although the exact mechanisms remain unclear, this finding highlights the potential ecological role of this compound in mediating interactions between fungi and termites [].
Disclaimer and Information on In-Vitro Research Products
Please be aware that all articles and product information presented on BenchChem are intended solely for informational purposes. The products available for purchase on BenchChem are specifically designed for in-vitro studies, which are conducted outside of living organisms. In-vitro studies, derived from the Latin term "in glass," involve experiments performed in controlled laboratory settings using cells or tissues. It is important to note that these products are not categorized as medicines or drugs, and they have not received approval from the FDA for the prevention, treatment, or cure of any medical condition, ailment, or disease. We must emphasize that any form of bodily introduction of these products into humans or animals is strictly prohibited by law. It is essential to adhere to these guidelines to ensure compliance with legal and ethical standards in research and experimentation.