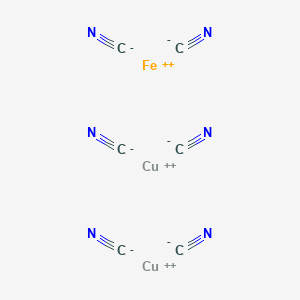
Cupric ferrocyanide
Overview
Description
Cupric ferrocyanide, also known as copper(II) ferrocyanide, is an inorganic compound with the chemical formula Cu2[Fe(CN)6]. It is a coordination compound consisting of copper and ferrocyanide ions. This compound is known for its distinctive reddish-brown color and is used in various applications, including analytical chemistry and industrial processes.
Preparation Methods
Synthetic Routes and Reaction Conditions: Cupric ferrocyanide can be synthesized through a precipitation reaction involving copper(II) salts and potassium ferrocyanide. The general reaction is as follows:
2Cu2++[Fe(CN)6]4−→Cu2[Fe(CN)6]
In a typical laboratory preparation, an aqueous solution of copper(II) sulfate is mixed with an aqueous solution of potassium ferrocyanide. The reaction occurs at room temperature, resulting in the formation of a reddish-brown precipitate of this compound.
Industrial Production Methods: Industrial production of this compound follows a similar approach but on a larger scale. The reaction is carried out in large reactors, and the precipitate is filtered, washed, and dried to obtain the final product. The purity and yield of the compound can be optimized by controlling the reaction conditions, such as concentration, temperature, and pH.
Chemical Reactions Analysis
Types of Reactions: Cupric ferrocyanide undergoes various chemical reactions, including:
Oxidation: this compound can be oxidized to form cupric ferricyanide.
Reduction: It can be reduced to form cuprous ferrocyanide.
Substitution: The ferrocyanide ion can undergo substitution reactions with other ligands.
Common Reagents and Conditions:
Oxidation: Oxidizing agents such as hydrogen peroxide or potassium permanganate can be used.
Reduction: Reducing agents like sodium borohydride or hydrazine are commonly employed.
Substitution: Ligands such as ammonia or ethylenediamine can be used for substitution reactions.
Major Products Formed:
Oxidation: Cupric ferricyanide.
Reduction: Cuprous ferrocyanide.
Substitution: Various substituted ferrocyanide complexes.
Scientific Research Applications
Cupric ferrocyanide has a wide range of scientific research applications, including:
Chemistry: It is used as a reagent in analytical chemistry for the detection and quantification of various ions and compounds.
Biology: this compound is used in histology for staining tissues and cells.
Industry: this compound is used in the purification of gases, removal of heavy metals from wastewater, and as a catalyst in chemical reactions.
Mechanism of Action
The mechanism of action of cupric ferrocyanide involves its ability to form stable complexes with various ions and molecules. The ferrocyanide ion acts as a ligand, coordinating with metal ions to form stable complexes. This property is utilized in various applications, such as ion exchange, catalysis, and analytical chemistry.
Molecular Targets and Pathways: this compound interacts with metal ions and other molecules through coordination bonds. The ferrocyanide ion can form stable complexes with transition metals, making it useful in various chemical processes.
Comparison with Similar Compounds
Cupric ferrocyanide can be compared with other similar compounds, such as:
Ferric ferrocyanide (Prussian blue): Known for its deep blue color and used as a pigment and in medical applications.
Nickel ferrocyanide: Used in electroplating and as a catalyst.
Zinc ferrocyanide: Employed in the purification of gases and as a stabilizer in the rubber industry.
Uniqueness: this compound is unique due to its reddish-brown color and its specific applications in analytical chemistry and industrial processes. Its ability to form stable complexes with various ions makes it a valuable compound in scientific research and industrial applications.
Properties
IUPAC Name |
dicopper;iron(2+);hexacyanide | |
---|---|---|
Source | PubChem | |
URL | https://pubchem.ncbi.nlm.nih.gov | |
Description | Data deposited in or computed by PubChem | |
InChI |
InChI=1S/6CN.2Cu.Fe/c6*1-2;;;/q6*-1;3*+2 | |
Source | PubChem | |
URL | https://pubchem.ncbi.nlm.nih.gov | |
Description | Data deposited in or computed by PubChem | |
InChI Key |
JGWCIFGWLIVWQZ-UHFFFAOYSA-N | |
Source | PubChem | |
URL | https://pubchem.ncbi.nlm.nih.gov | |
Description | Data deposited in or computed by PubChem | |
Canonical SMILES |
[C-]#N.[C-]#N.[C-]#N.[C-]#N.[C-]#N.[C-]#N.[Fe+2].[Cu+2].[Cu+2] | |
Source | PubChem | |
URL | https://pubchem.ncbi.nlm.nih.gov | |
Description | Data deposited in or computed by PubChem | |
Molecular Formula |
C6Cu2FeN6 | |
Source | PubChem | |
URL | https://pubchem.ncbi.nlm.nih.gov | |
Description | Data deposited in or computed by PubChem | |
DSSTOX Substance ID |
DTXSID70929219 | |
Record name | Copper(2+) iron(2+) cyanide (2/1/6) | |
Source | EPA DSSTox | |
URL | https://comptox.epa.gov/dashboard/DTXSID70929219 | |
Description | DSSTox provides a high quality public chemistry resource for supporting improved predictive toxicology. | |
Molecular Weight |
339.04 g/mol | |
Source | PubChem | |
URL | https://pubchem.ncbi.nlm.nih.gov | |
Description | Data deposited in or computed by PubChem | |
Physical Description |
Reddish-brown solid; [Merck Index] | |
Record name | Cupric ferrocyanide | |
Source | Haz-Map, Information on Hazardous Chemicals and Occupational Diseases | |
URL | https://haz-map.com/Agents/9056 | |
Description | Haz-Map® is an occupational health database designed for health and safety professionals and for consumers seeking information about the adverse effects of workplace exposures to chemical and biological agents. | |
Explanation | Copyright (c) 2022 Haz-Map(R). All rights reserved. Unless otherwise indicated, all materials from Haz-Map are copyrighted by Haz-Map(R). No part of these materials, either text or image may be used for any purpose other than for personal use. Therefore, reproduction, modification, storage in a retrieval system or retransmission, in any form or by any means, electronic, mechanical or otherwise, for reasons other than personal use, is strictly prohibited without prior written permission. | |
CAS No. |
13601-13-3 | |
Record name | Cupric ferrocyanide | |
Source | ChemIDplus | |
URL | https://pubchem.ncbi.nlm.nih.gov/substance/?source=chemidplus&sourceid=0013601133 | |
Description | ChemIDplus is a free, web search system that provides access to the structure and nomenclature authority files used for the identification of chemical substances cited in National Library of Medicine (NLM) databases, including the TOXNET system. | |
Record name | Copper(2+) iron(2+) cyanide (2/1/6) | |
Source | EPA DSSTox | |
URL | https://comptox.epa.gov/dashboard/DTXSID70929219 | |
Description | DSSTox provides a high quality public chemistry resource for supporting improved predictive toxicology. | |
Record name | Dicopper hexacyanoferrate | |
Source | European Chemicals Agency (ECHA) | |
URL | https://echa.europa.eu/substance-information/-/substanceinfo/100.033.695 | |
Description | The European Chemicals Agency (ECHA) is an agency of the European Union which is the driving force among regulatory authorities in implementing the EU's groundbreaking chemicals legislation for the benefit of human health and the environment as well as for innovation and competitiveness. | |
Explanation | Use of the information, documents and data from the ECHA website is subject to the terms and conditions of this Legal Notice, and subject to other binding limitations provided for under applicable law, the information, documents and data made available on the ECHA website may be reproduced, distributed and/or used, totally or in part, for non-commercial purposes provided that ECHA is acknowledged as the source: "Source: European Chemicals Agency, http://echa.europa.eu/". Such acknowledgement must be included in each copy of the material. ECHA permits and encourages organisations and individuals to create links to the ECHA website under the following cumulative conditions: Links can only be made to webpages that provide a link to the Legal Notice page. | |
Record name | CUPRIC FERROCYANIDE | |
Source | FDA Global Substance Registration System (GSRS) | |
URL | https://gsrs.ncats.nih.gov/ginas/app/beta/substances/871D97G82Z | |
Description | The FDA Global Substance Registration System (GSRS) enables the efficient and accurate exchange of information on what substances are in regulated products. Instead of relying on names, which vary across regulatory domains, countries, and regions, the GSRS knowledge base makes it possible for substances to be defined by standardized, scientific descriptions. | |
Explanation | Unless otherwise noted, the contents of the FDA website (www.fda.gov), both text and graphics, are not copyrighted. They are in the public domain and may be republished, reprinted and otherwise used freely by anyone without the need to obtain permission from FDA. Credit to the U.S. Food and Drug Administration as the source is appreciated but not required. | |
Retrosynthesis Analysis
AI-Powered Synthesis Planning: Our tool employs the Template_relevance Pistachio, Template_relevance Bkms_metabolic, Template_relevance Pistachio_ringbreaker, Template_relevance Reaxys, Template_relevance Reaxys_biocatalysis model, leveraging a vast database of chemical reactions to predict feasible synthetic routes.
One-Step Synthesis Focus: Specifically designed for one-step synthesis, it provides concise and direct routes for your target compounds, streamlining the synthesis process.
Accurate Predictions: Utilizing the extensive PISTACHIO, BKMS_METABOLIC, PISTACHIO_RINGBREAKER, REAXYS, REAXYS_BIOCATALYSIS database, our tool offers high-accuracy predictions, reflecting the latest in chemical research and data.
Strategy Settings
Precursor scoring | Relevance Heuristic |
---|---|
Min. plausibility | 0.01 |
Model | Template_relevance |
Template Set | Pistachio/Bkms_metabolic/Pistachio_ringbreaker/Reaxys/Reaxys_biocatalysis |
Top-N result to add to graph | 6 |
Feasible Synthetic Routes
Disclaimer and Information on In-Vitro Research Products
Please be aware that all articles and product information presented on BenchChem are intended solely for informational purposes. The products available for purchase on BenchChem are specifically designed for in-vitro studies, which are conducted outside of living organisms. In-vitro studies, derived from the Latin term "in glass," involve experiments performed in controlled laboratory settings using cells or tissues. It is important to note that these products are not categorized as medicines or drugs, and they have not received approval from the FDA for the prevention, treatment, or cure of any medical condition, ailment, or disease. We must emphasize that any form of bodily introduction of these products into humans or animals is strictly prohibited by law. It is essential to adhere to these guidelines to ensure compliance with legal and ethical standards in research and experimentation.